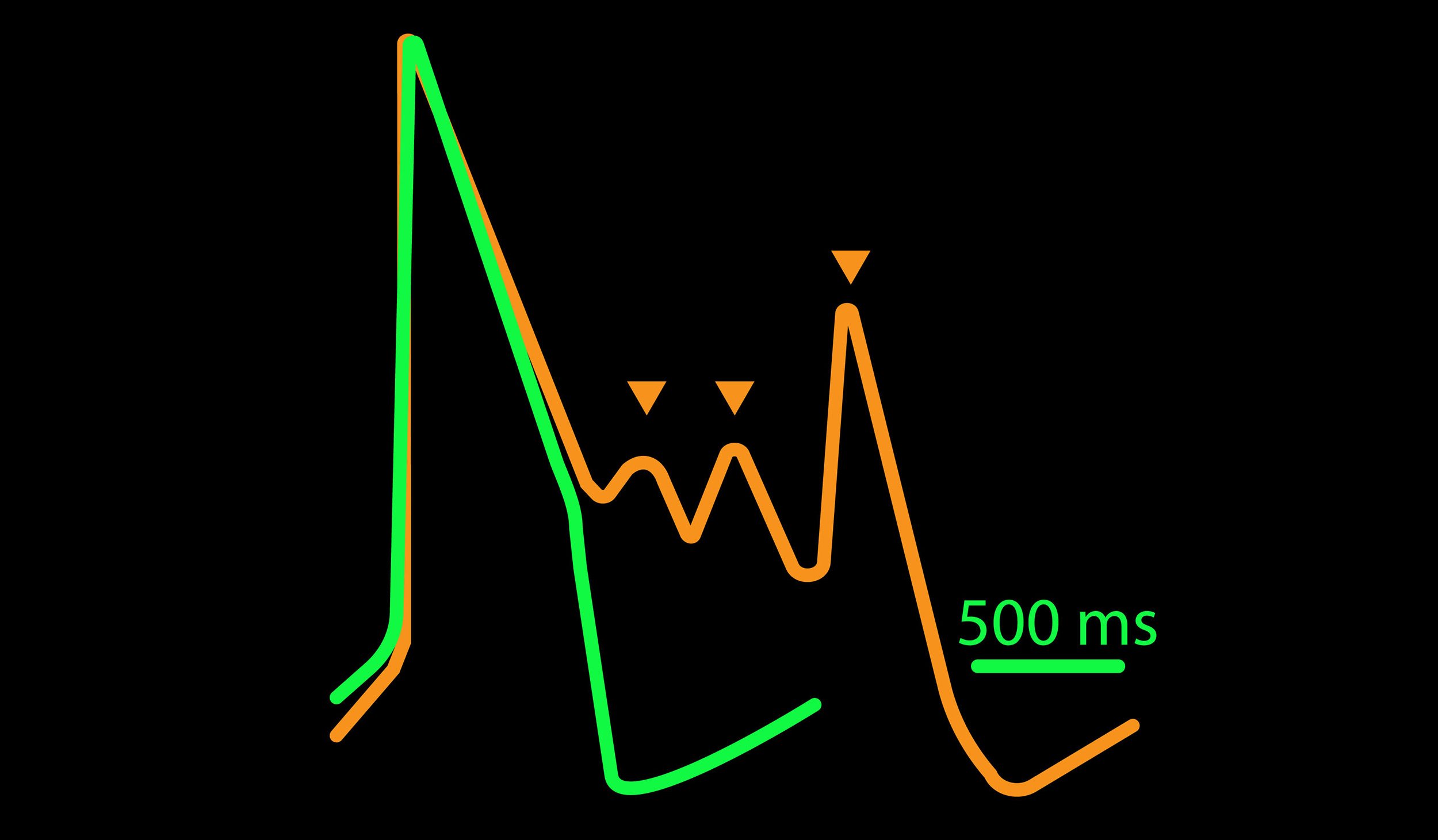
Human stem-cell derived cardiomyocyte MEA assays can screen for pro-arrhythmic effects. These models enable real-time monitoring of functional electrophysiological endpoints that parallel clinical safety markers.
The inability to predict the cardiovascular liability of therapeutic compounds prior to clinical trials has resulted in numerous costly late-stage drug development failures and market withdrawals leading to the development of New Approach Methodologies (NAMs).
Providing deep insights into human in vitro models, the Maestro MEA platform is a vital tool for any cardiac safety testing paradigm.
Translatable human in vitro cardiac assay
MEA technology is a powerful tool for predicting cardiac risk.
Analogous to an in vivo EKG signal, an MEA field potential can measure changes in beating, depolarization and repolarization. But the Maestro MEA platform is more than MEA:
- Arrhythmia detection
Maestro MEA is the only MEA platform capable of LEAP™ action potential analysis. - Propagation alterations
Propagation issues can lead to deadly re-entrant currents. MEA is the best platform for evaluating propagation changes in vitro. - Contractility assessment
Measuring both electrical and contractile properties across the culture. - Viability multiplexing
Maestro MEA can simultaneously measure viability and function.
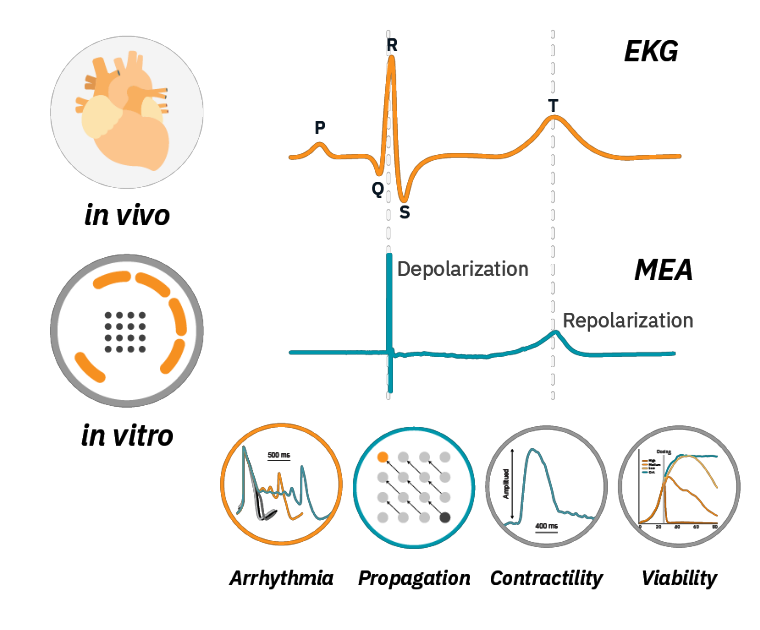
Real-time, label-free in vitro cardiac analysis
-
Highly reproducible and predictive in vitro cardiotoxicity assay>
-
Measure real-time, label-free in vitro cardiac activity>
-
Detect subtle changes in action potential morphology>
-
Assay steps: Human iPSC-derived cardiomyocytes>
-
Establishing a functional standard for stem-derived cardiomyocytes>
-
Application Note: Development of a functional cardiotoxicity assay for evaluating the safety of cell therapies>
-
Track propagation across the cardiac syncytium>
-
Elicit mature force-frequency responses with chronic pacing>
The Maestro MEA cardiac activity assay has been proven to be highly accurate with low variability. In a multi-site, blinded study to assess proarrhythmic risk of cardioactive compounds, Maestro MEA outperformed the competition in a head-to-head comparison.
| Maestro MEA | MEA2100 | xCELLigence RTCA CardioECR |
Most Reliable Sites that passed QC | 85% | 42% | 25% |
Most Accurate Identified compounds expected to induce a 20% change in FPDc | 93% | 50% | 75% |
Lowest Variability Well-to-well FPDc variability in baseline | 6.4% | 21.5% | 15.2% |
The study (Millard et al, 2018) investigated 8 compounds in 18 studies across 13 global sites, 3 MEA platforms, and 4 hiPSC cardiomyocyte sources. 85% of Maestro MEA sites passed initial QC, more than double the next MEA system.
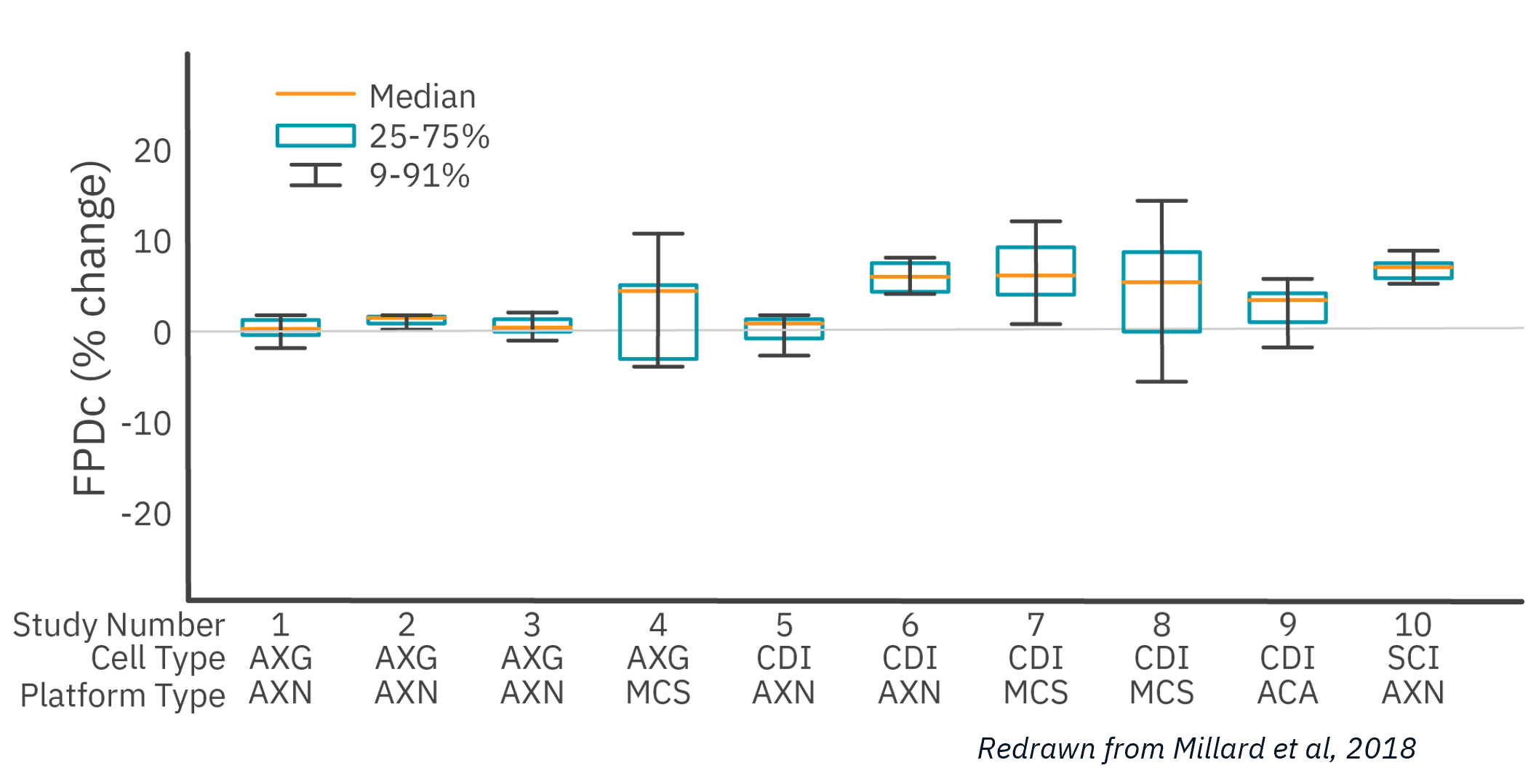
Of the 10 studies that passed QC acceptance criteria, Maestro MEA had the lowest well-to-well variability, 58% less than the next lowest despite being performed across 6X as many sites.
The Maestro MEA assay correctly identified test compounds 93% of the time. A follow-up study (Blinova et al 2018) expanded the scope to 28 blinded compounds of low, intermediate, and high risk. Maestro MEA had a 0.93 correlation coefficient between study sites.
With these studies, together with key opinion leaders in academia and industry, Axion has developed a proposed standard for hiPSC cardiomyocytes.
Measure key metrics of cardiac depolarization, repolarization, beating and arrhythmia. Cardiac field potentials can be measured label-free in real time using Maestro MEA. The method is noninvasive and requires only basic cell culturing technique allowing for long-term monitoring of cultures, or acute drug responses.
To demonstrate MEA’s ability to characterize cardiac activity, human iPSC-derived cardiomyocytes were dosed with cardioactive compounds.
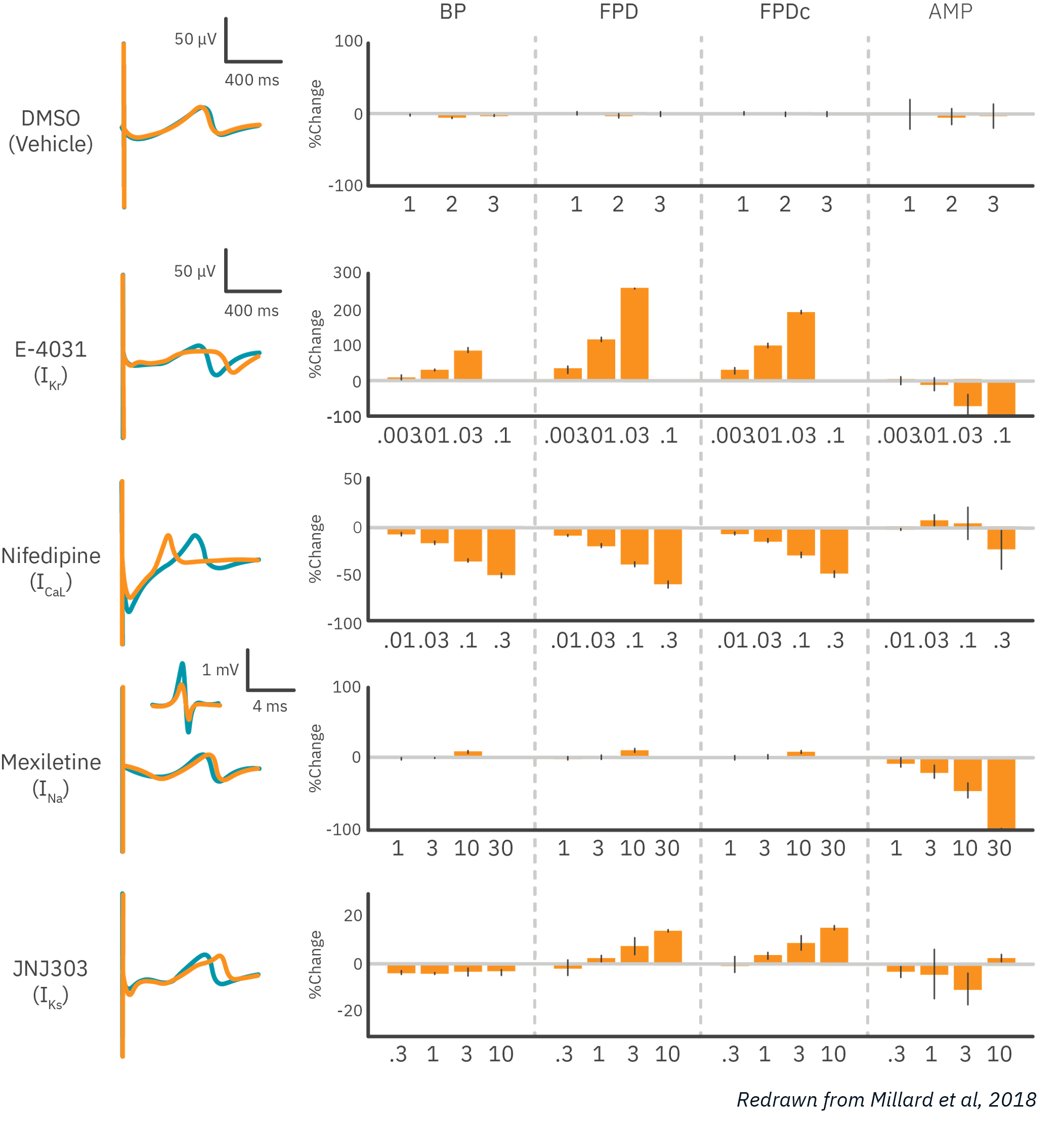
Results: MEA was able to detect dose-dependent effects of compounds targeting major cardiac ion channels. As expected, potassium channel block prolonged field potential duration (FPD), while calcium channel block shortened FPD, and sodium channel block reduced the amplitude of the depolarization. Data courtesy of Millard et al, 2018 and CiPA.
Cardiac action potentials are the culmination of complex interactions that orchestrate the electrical activity of the heart. LEAP (local extracellular action potential), a unique capability of Maestro MEA, can quantify action potential morphology and detect subtle changes to biology with the best signal:noise ratio. Key metrics, such as rise time, action potential duration (APD), triangulation ratio, and percentage of beats with EADs are all automatically detected.
To demonstrate LEAP’s ability to characterize action potential morphology in vitro, human iPSC-derived cardiomyocytes were dosed with cardioactive compounds.
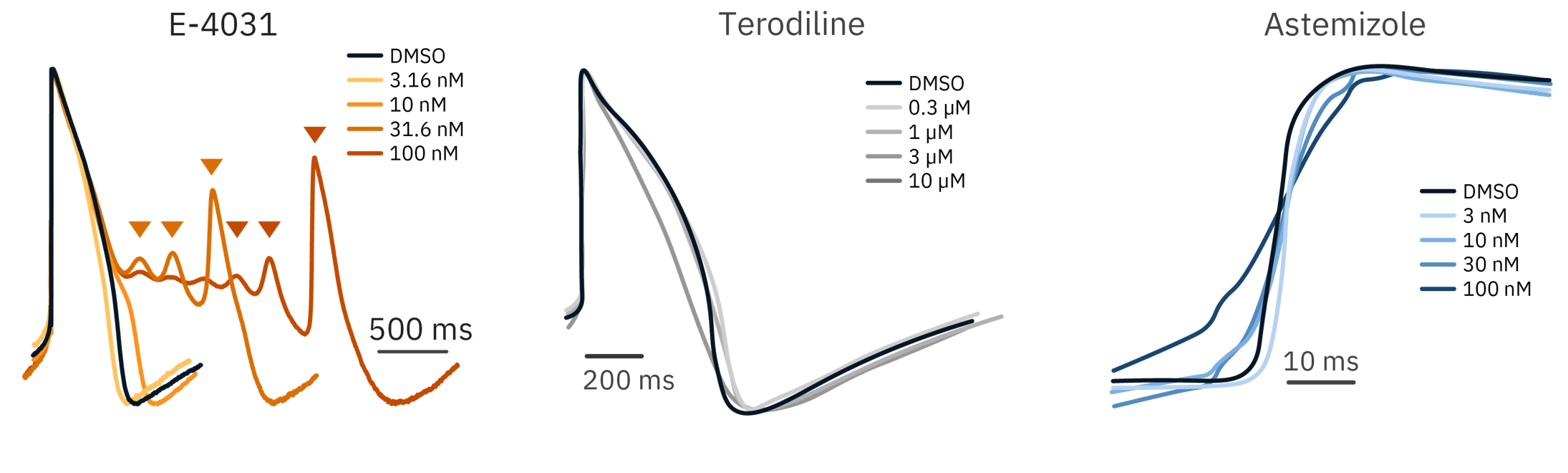
Results: LEAP was able to detect action potential changes indicative of cardiotoxicity: slowing depolarization, waveform triangulation, and early after depolarizations (EADs). Each compound responded in a dose-dependent manner.
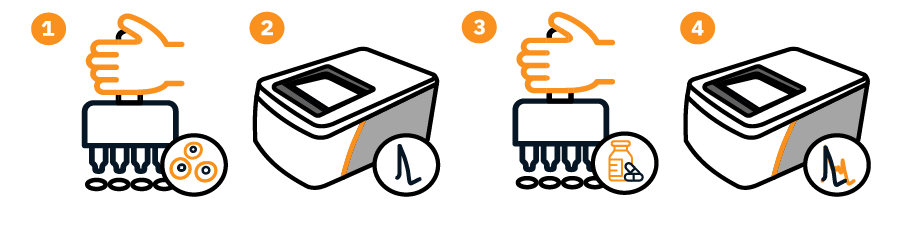
The Maestro MEA is ideal for measuring cardiotoxicity in human iPSC-derived cardiomyocytes and modeling proarrhythmic risk. Simply (1) plate and culture the cardiomyocytes normally on a CytoView MEA plate. (2) Record a baseline. (3) Add compound. (4) Record compound effects.
Download these cardiomyocyte protocols for leading stem cell-derived providers:
- >> fCDI iCell Human iPSC-derived cardiomyocyte protocol
- >> fCDI iCell Cardiomyocytes2 application protocol
- >> AXOL axoCELLS user guide: Human iPSC-derived atrial and ventricular cardiomyocytes on the Axion Maestro MEA
- >> Cardiac safety applications using Axiogenesis Cor.4U cardiomyocytes and the Maestro MEA platform
- >> Cardiac safety assessment during electrical pacing using pluricyte cardiomyocytes and e-stim + MEA plates
Together with key opinion leaders in academia and in industry, and combined with published data, we developed a proposed standard for hiPSC cardiomyocyte functionality on MEA. Briefly, this standard focuses on the hiPSC-cardiomyocyte spontaneous beat rate, features of the cardiac waveform (depolarization spike amplitude and field potential duration), and the synchronization of activity in the syncytia.
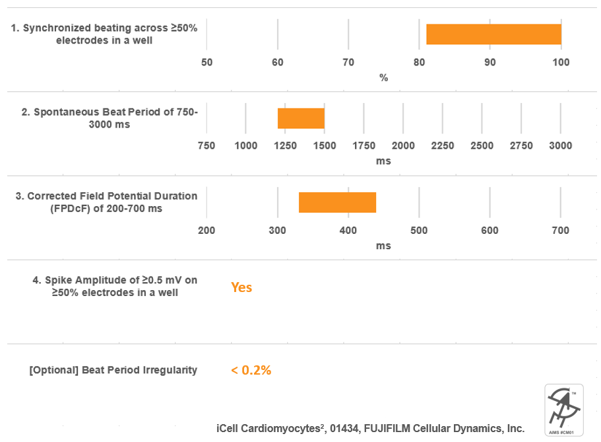
An example of cell performance data from a leading commercial source of hiPSC-cardiomyocytes with respect to this proposed standard.
Want to read the full standard and learn more about Axion iPSC Model Standards (AIMS), including self-certified vendors, recommended protocols, and selected publications?
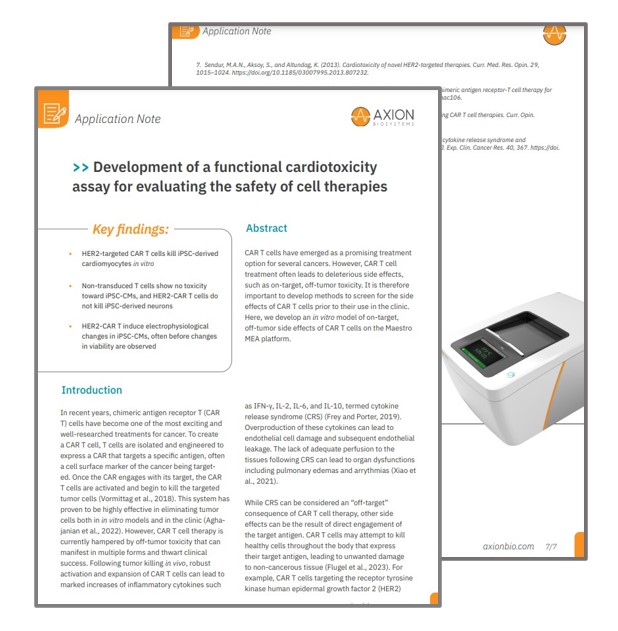
In this app note you will learn how:
>> HER2-targeted CAR T cells kill iPSC-derived cardiomyocytes in vitro
>> Non-transduced T cells show no toxicity toward iPSC-CMs, and HER2-CAR T cells do not kill iPSC-derived neurons
>> HER2-CAR T induce electrophysiological changes in iPSC-CMs, often before changes in viability are observed
Coordinated propagation is critical to heart function but requires simultaneous measurements from multiple locations on the cardiac syncytium. With an array of sensitive electrodes, Maestro MEA is the ideal tool for assessing conduction velocity and propagation patterns.
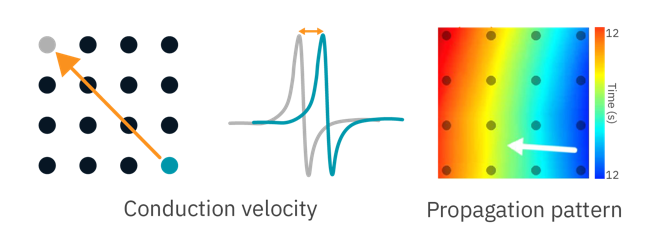
To demonstrate the Maestro MEA’s propagation assay, human iPSC-derived cardiomyocytes were dosed with cardioactive compounds.
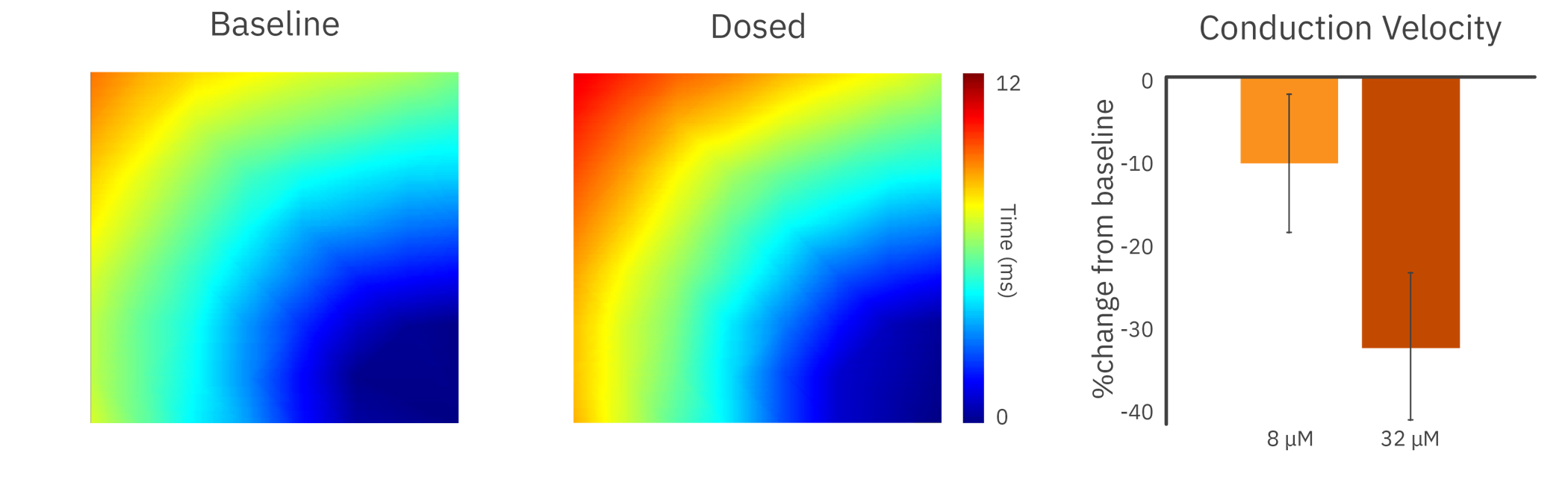
In the presence of a sodium channel blocker, the cardiomyocytes showed a decrease in conduction velocity across the array.
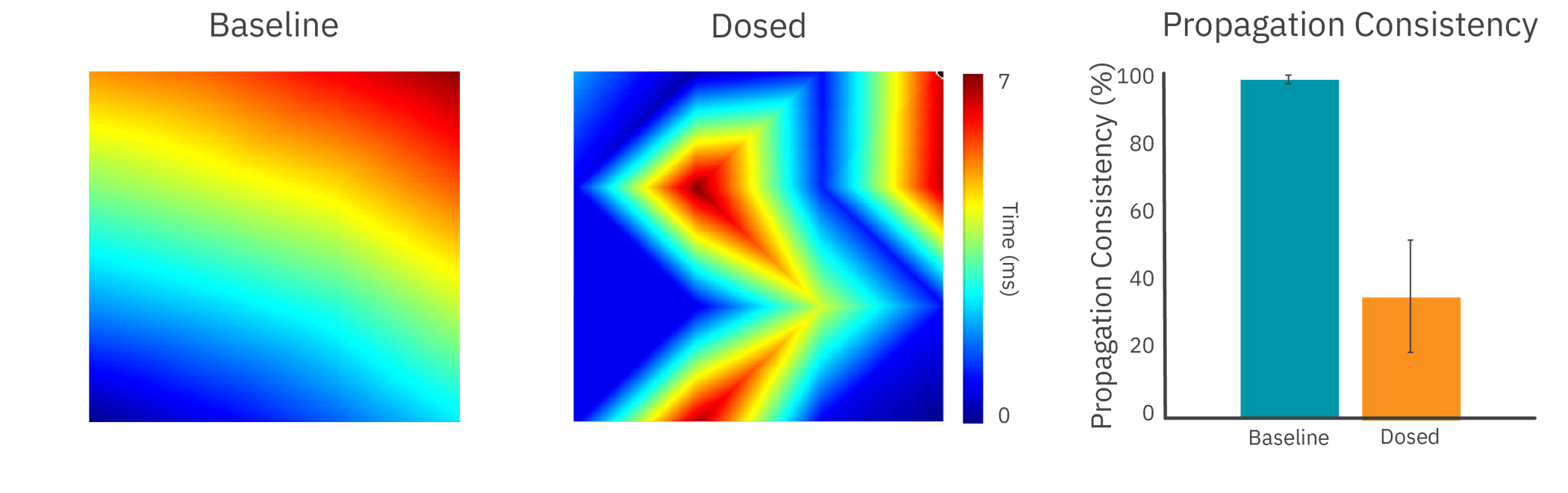
The addition of an anticancer compound disrupted propagation across the syncytium, altering the propagation pattern and reducing propagation consistency.
Results: With high sampling rates across an array of electrodes, Maestro MEA was able to detect slowing and disruption of cardiac propagation across the culture.
Purpose: To elicit a mature force-frequency relationship in hiPSC-derived cardiomyocytes. In a mature cardiomyocyte, an increase in frequency is accompanied by an increase in contractile strength. This force-frequency relationship is often lacking in hiPSC-derived cardiomyocytes and is a sign of their immaturity.
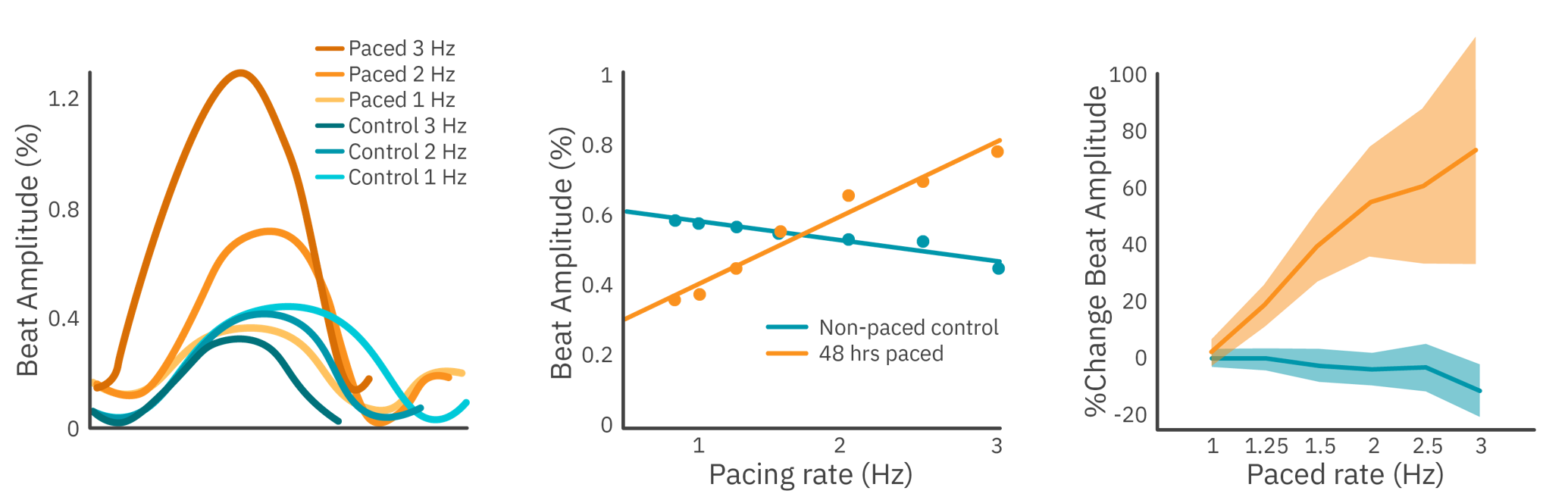
Electrical stimulation was used to measure the force-frequency relationship of hiPSC-derived cardiomyocytes with the Maestro MEA. Prior to testing, the culture was kept on the Maestro MEA for 48 hours, chronically pacing half of the wells.
Results: Cardiomyocytes chronically paced for 2 hours demonstrated a mature force-frequency relationship as measured by beat amplitude using Maestro contractility.
Want to learn how?
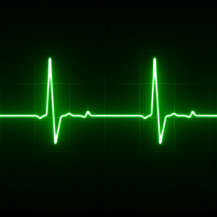
Cardiac Metric Definitions
Discover our Cardiac Metrics Definition guide to discover the full list of metrics available in our cardiac analysis tool.