What you will learn in this on-demand cell therapy webinar:
>> Current status of CAR T cell therapy for solid tumors
>> How to optimize CAR structure during immunotherapy development for enhanced efficacy
>> Using the Axion Omni FL and Omni Pro 12 live-cell imaging platform to evaluate potency of cell therapies in vitro
About the presenters:
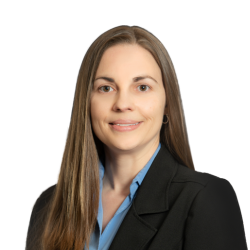
Stacie Chvatal, PhD
Director of Product Management at Axion BioSystems
Dr. Stacie Chvatal is the Director of Product Management at Axion BioSystems. She earned a Bachelor of Science in Engineering from Mercy University, and a PhD in Biomedical Engineering from the Georgia Institute of Technology and Emory University.
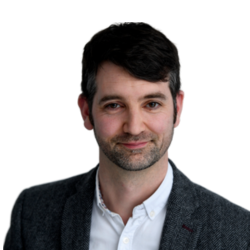
Marc Davies, PhD
Vice President of R&D at Leucid Bio
Dr. Marc Davies received his PhD from the laboratory of Dr. Joe Maher at King's College London, where his research focused on the development and pre-clinical assessment of CAR T cells targeting the extended RB family.
Transcript of webinar:
Zhong Yu: Hello, everyone, and welcome to today's webinar, "Optimizing CAR Structures to Enhance Efficacy in Solid Tumors: Moving from Linear to Lateral Architectures." I am Zhong Yu, the Scientific Relations Manager of Axion BioSystems, and I'll be your moderator for today's event.
Today's educational web seminar is presented by Lab Roots and brought to you by Axion BioSystems. We encourage you to participate today by submitting any questions you may have during the presentation. To do so, simply type them into the "Ask a Question" box and click "Send." We'll answer as many questions as we have time for at the end of the presentation. You may also submit any technical issues here as well.
I'd like to welcome our speakers, Dr. Marc Davies and Dr. Stacie Chvatal. Marc received his PhD from the laboratory of Dr. Joe Maher at King's College London, where his research focused on the development and pre-clinical assessment of CAR T cells targeting the extended RB family. Dr. Chvatal is the Director of Product Management at Axion BioSystems. Stacie earned her bachelor's degree in Science in Engineering from Mercer University and her PhD in Biomedical Engineering from the Georgia Institute of Technology and Emory University. For a complete biography of today's speakers, click on their names in the presenters' window at the top right of your screen.
We will begin with Stacie. Stacie, you may now begin your presentation.
Stacie Chvatal: Hi, everyone, and thank you for joining us for the webinar today. Soon, you'll hear from Dr. Davies about his work in optimizing CAR structures to better target solid tumors. But first, I'd like to introduce you to Axion and some of our live cell analysis tools that can help accelerate immunotherapy development. I'm Stacie Chvatal, and I'm the Director of Product Management here at Axion.
In case you aren't familiar with Axion BioSystems, the company was founded in 2008 in Atlanta, Georgia, and now has four office locations and over 170 employees worldwide. Our first products were Microelectrode Array (MEA) instruments that are used to study electrically active cells, so we're pretty well-established in neural and cardiac disease modeling and drug discovery. We later expanded our electro-based technology to impedance measurements and platforms that are used to study cell proliferation, cytotoxicity, and more in any cell type. Most recently, we expanded the portfolio to include live-cell imaging instruments and can now address a wide range of applications, including cancer and beyond. We now have over 2500 systems in the field with placements at more than 60 immuno-oncology and cell and gene therapy companies. There are more than 800 peer-reviewed publications using Axion technology, and our Omni whole plate imaging platform alone has been used for over two hundred thousand scans in just a few years since launch.
We've seen in recent years that immunotherapy has great promise for treating cancer and has changed how we intervene in disease. Immuno-oncology approaches have dramatically improved patient outcomes. Here are some examples:
- Keytruda is an anti-PD1 monoclonal antibody or checkpoint inhibitor therapy that was first approved in 2014. Former President Jimmy Carter received it as treatment for melanoma that had metastasized.
- Kymriah is the first FDA-approved cell therapy used to treat pediatric ALL. Emily Whitehead was the first patient to receive this therapy back in 2012 when the product was still in the phase one clinical trial, and she remains cancer-free to this day.
As these therapies continue to evolve and improve, there's also a need for improved analytical tools and more predictive live-cell in vitro assays. Axion has a suite of easy-to-use tools that you can use to quantify cell function label-free and in real-time.
First, I'll tell you a little about the Maestro impedance-based platforms, and after that, I'll talk briefly about the Omni live-cell imaging products.
The Maestro Z range includes benchtop instruments Maestro Z and ZHT, which have built-in environmental control and a multi-plate Maestro tray system that goes inside the incubator and accommodates up to eight plates. All of these systems have compliance features built in, so they can grow with your program.
The Maestro Z products are based on impedance measurement. They use multi-well plates that have sensitive electrodes embedded in the substrate. You can culture cells in the plate just as you would a standard tissue culture plate. To measure the impedance, very small electrical signals are passed between electrodes. When there are no cells in the well, the electrical current passes easily, and impedance is low. When cells are present and attached to the substrate, they block these electrical currents, and you see an increase in impedance. If a drug or cell therapy causes the cells to die or detach, the impedance will decrease. The impedance assay can be used to study cell proliferation and viability, cytotoxicity of chemotherapy agents and cell therapies, cell migration, strength of cell-to-cell coupling, and more. It's used in a number of different application areas, including immuno-oncology.
Because the impedance measurement is non-invasive and label-free, you can continuously monitor the dynamics of the changes in real-time over minutes, hours, or days without disturbing the underlying biology.
Here's an example from a recent paper where the researchers were studying CAR T cell killing in glioblastoma. GD2 is abundantly expressed in human glioblastoma samples. In this study, the authors compared retrovirally transduced GD2-targeted CAR T cells to CRISPR-edited or VFC CAR T cells. The CAR T cell products were co-cultured with patient-derived glioma stem cells at three different effector-target ratios, and impedance was monitored continuously for seven days.
At the seven-day endpoint, the retroviral and CRISPR-edited CAR T cells showed similar cytotoxicity. However, when you look at the time course of the cell killing and the continuous plot, as well as the kinetic endpoints such as kill time 50, shown here, or the time required to reach 50 cytolysis, you can see that the VFC CAR T cells killed the target glioma cells much faster than the retroviral CAR T cells did. This illustrates the importance of kinetic data recording by impedance that's often missed with endpoint analysis.
Axion's Omni live-cell imaging platforms also let you monitor cells continuously from right inside your incubator. The Omni has a light source on top with an inverted microscope on the bottom. The open design allows you to image a variety of vessels, including well plates, flasks, microfluidic chips, and more. As you can see here, the Omni fits inside an incubator with plenty of space to spare and has automated acquisition and analysis so you can monitor your cells with ease anywhere, anytime.
To use Omni, you just put the vessel on the scanning bed, and the arm moves back and forth, taking images of the cells to create a whole vessel bright-field scan or fluorescent snapshots. The Omni is available in bright-field only and fluorescence models, both of which have automated acquisition, whole vessel bright-field scanning, and whole-well analysis. The Omni FL also has two fluorescence channels: red and green. With Axion's portal, you can access your images and do analysis from anywhere. Four software modules are available, including confluency, scratch assay, clonogenic assay, and organoid analysis.
The newest Omni instrument is the Omni Pro 12, which integrates a 12-plate hotel and robotic arm with the Omni so you can measure up to 12 plates. Each plate sits in a holder in the hotel, and the robotic arm in the back picks up the plate and moves it to the Omni surface to take a scan. Omni Pro 12 has flexible scheduling software, which makes it easy for multiple people to use the system for multiple applications. The whole system fits inside the incubator, so the cells stay in their optimal environment throughout the experiments.
You can use the Omni live-cell Imaging platforms for a variety of research applications, as you can see in this slide here. I'll focus on cancer and immuno-oncology applications.
First, you can use the Omni to measure transduction efficiency and optimize transduction conditions. In this example, the Omni FL was used to compare different transduction parameters, including baculo-virus vector concentration. This top plot shows the percentage of GFP-expressing cells over time in HeLa cells transduced with different virus particle per cell concentrations. As expected, the transduction rate increases with increasing virus concentration. However, a parallel analysis of cell proliferation that was done using the bright-field Confluence measurement shows that although the cells in all conditions proliferated equally to begin with, after 18 hours, the higher concentrations of virus had an adverse effect on the cell's proliferation. Ultimately, the most optimal conditions were 40 PPC and 20,000 cells per well, which is what you see in the images here on the right, resulting in high GFP expression and low toxicity.
Omni's continuous monitoring makes it easy to optimize multiple steps of a transduction procedure.
The Omni can also be used to monitor cell-mediated killing. In this example, target cells were transfected with GFP, and effector cells were stained with cell tracker Orange. The effector cells were added at a 10-to-1 ratio to the target cells. In this video, you can see the T cells clustering around the target cells, and by the end, there are many dead target cells with only debris remaining. That data is quantified here; the effector cells were added six hours after seeding the target cells. A decrease in green signal can be used as an indicator of the target cell death. These plots show green confluency and green object count; in both, it's clear that there's a dose-dependent decrease in target cell proliferation as a result of the effector cell killing. At the highest ratio, the target cells were killed completely.
3D cancer spheroids are promising models for studying solid tumors, which have unique challenges. With the Omni, you can continuously monitor cancer spheroids and track the effects of compounds on your cells over time. In this example, fluorescence was used to monitor the absorption of doxorubicin, which is a chemotherapy drug, into a cancer spheroid made from C6 glioma cells. As the drug penetrates the spheroid, red fluorescence increases. When higher concentrations of doxorubicin are used, more of the drug is able to penetrate the spheroid and reach the cells on the inside. With Omni, you can measure the amount and rate of drug absorption into spheroids to improve drug delivery.
In this final example, I'm showing immune cell-mediated killing using cancer spheroid targets. This video is from a collaboration with a company called 4D cell. 4D cell has special plates containing hydrogel-based micro-well structures that are used to form many spheroids in a single well, each one in its own micro-well. Here, you can see cancer spheroids in green and immune cells in red. The immune cells start to attack the cancer spheroids, leading to cell death, which can be measured by green intensity.
As I've shown here, Axion's live cell analysis tools make it easy to quantify cell function in real time.
Now I'll turn the presentation over to Dr. Marc Davies from Leucid Bio. Thanks for being here and sharing your work. Dr. Davies will present his recent work on optimizing CAR structures to enhance efficacy in solid tumors and show how Omni FL and Omni Pro 12 help in evaluating the potency of cell therapies.
Marc Davies: So, before we get into some of the data, let me share a bit about Leucid Bio. I'm Marc Davies, the VP of R&D at Leucid Bio. I'd like to start by thanking Axion for inviting me here today to talk about the work that we're doing to optimize CAR structures and enhance the efficacy of CAR T-cell therapy in solid tumors, moving from linear to lateral architectures.
A little bit about Leucid Bio: We are an early-phase CAR T-cell company based at Guy's Hospital in London. In fact, we're almost a clinical-stage company now, with our first assets due to enter trials in the coming months. Our primary focus is on the development of CAR T cells for the treatment of solid tumors. CAR T cells have made their name in the treatment of hematological malignancies, where their efficacy has been transformative in the treatment of these diseases. There are now seven approved therapies for B-cell and plasma cell malignancies. However, there's no equivalent in solid tumors as yet, despite the fact that there is a much higher unmet need. Many of these challenges arise from the unique difficulties in targeting solid tumors.
The work we've done at Leucid is founded on over 20 years of pioneering research from the laboratory of Dr. John Marr at King's College London. John is also Leucid's Chief Scientific Officer and scientific founder. He was the first to engineer and develop CD-28-based second-generation CARs in human primary T cells, following on from the seminal work done by Helen Finney in Jurkat cells back in the late '90s.
Within Leucid, we have a burgeoning pipeline of assets. Much of what I'll talk about today involves novel CAR structure platforms that we've developed to enhance the baseline efficacy of CAR T cells. In addition to this, we're also working on a number of novel armoring technologies to further enhance the efficacy of our T cells. We've developed unique manufacturing processes to achieve robust generation of drug products while minimizing the impact on patients themselves. We do all of this in both autologous and allogeneic chassis as a way of future-proofing our technology.
So, I've already mentioned that targeting solid tumors with CAR T cells is fraught with a number of additional hurdles that are distinct from the hematological space. Some of these challenges are admittedly matched with the hematological space, including things such as antigen loss. However, within solid tumors, antigen heterogeneity is a significant issue. In fact, the scarcity of suitable antigens that you can target—those that are present on the tumor but not on healthy vital organs—is much more challenging than when targeting B cells or plasma cells. And, of course, the big challenge is the immunosuppressive tumor microenvironment, which is much more prevalent within the solid tumor space.
This schematic I find very important and forms the cornerstone of everything we do at Leucid. To have confidence that your therapy will benefit patients, you need to meet at least the majority of these challenges.
Now, let's take a step back and discuss what we're currently doing based on our own clinical experience. We have an ongoing academic clinical trial run by Dr. Morris, the chief investigator, in patients with relapsed and refractory head and neck cancer. We target this using a second-generation CAR known as T4. T4, instead of using an antibody as the targeting moiety, uses a peptide—a chimeric peptide, to be precise. It's formed from a fusion of two EGFR-specific ligands, TGF Alpha and EGF. When combined to form the tie peptide, they lose their binding specificity for just EGFR and become more promiscuous.
What this allows us to do is target the entire HERB superfamily, including HER2, HER3, and HER4. In fact, we've shown we can target eight out of nine of the homo- or hetero-dimers formed by this superfamily. The reason for this approach is the tumor's ability to become resistant to targeted therapies, especially in head and neck cancer. Patients often receive cetuximab, an EGFR-specific antibody, and while many initially respond, resistance often develops as the tumor cells down-regulate EGFR and up-regulate other members of the EGFR superfamily—particularly the HER2-HER3 heterodimer. By using a peptide with this binding promiscuity, we're able to address both antigen heterogeneity and evolved resistance that tumors develop to other targeted therapies.
Alongside the second-generation CAR, we've co-expressed a second receptor known as T4 Alpha Beta. This is a fusion protein in which the R4 receptor Alpha is a fusion domain fused directly in frame with the R2 IL-15 receptor beta chain. What this does is, when we give the T cells what's otherwise a very poor mitogen, interleukin-4 Alpha Beta chain is able to complex with the common gamma chain, resulting in a stat five signal similar to that achieved with interleukin-2. So we trick the T cells into thinking they're seeing interleukin-2, whereas in fact, they're seeing interleukin-4. The reason for this was we are treating end-stage patients, and we weren't sure how well we would be able to transduce their T cells. And so the interleukin-4 allows us to selectively enrich for the transduced population of cells because they're the only ones that can proliferate in response to interleukin-4.
Now, the extended OB family is expressed on a number of vital organs within the body, and therefore we de-risked going after this target, again ticking the box of the paucity of suitable antigens by undertaking an intra-tumoral administration of these T cells, i.e., directly injecting the T cells into the target lesion themselves. And this is just an image from our clinical trial in which we've done passive labeling with radioactive indium to show that the T cells, once injected into the lesion, do not leak out again.
So if we go back to our schematic, we're already starting to tick a number of these boxes. We've overcome the issue of the suitable antigens by going after things that are present in other organs in the body, but we're restricting the access of our car T cells exclusively to the tumor. We've overcome the issue of antigen heterogeneity and antigen loss by making sure our car is able to bind multiple targets, and we're cheating by overcoming trafficking to the tumor site by simply injecting the T cells directly in.
What we were less sure about is how persistent these T cells would be. While they show good pre-clinical efficacy, how efficacious would they be in the patient? Do they maintain persistence and functional persistence? So what I would say is that we've now treated 19 patients to date, and we've seen no dose-limiting toxicities. We've also had no batch failures, and the reason I bring this up is we have a unique manufacturing process that comes from whole blood, not from a leuko foresate. Now, this is done with two reasons in mind. Firstly, these patients, when they come onto study, are often rapidly progressing, and we want to speed up the ability to get our therapy to these patients. As a result, we don't want to have to wait to schedule a leukocytapheresis, and so we've developed our manufacturing process from whole blood. In addition to this, a number of our patients are too frail to sit on a leukopheresis machine. So, again, going down the route of whole blood provides us with the speed to get these therapies to the patients while also minimizing the impact upon them themselves. The product is also injected as a fresh product, so the vein-to-tumor time is no longer than 12 days.
In terms of efficacy, as I mentioned, our patients are rapidly progressing on entry into the study, and most of them achieve stable disease by six weeks post-treatment. CD19 Castle CAR T-Cell Therapy and BCMA CAR T-Cell Therapy are not, but we are having an impact on our patients' lives, as shown by this intention-to-treat analysis.
The red arrow represents the median survival of patients where they would be expected to be in the absence of treatment with T4, whereas the black arrow shows the 100% mortality rate had they not received T4 based on the historical data for this cohort of patients. You can see we've extended the survival of these patients far beyond that.
However, it is true that what we now need to do is work out how to turn these stable diseases into partial responses and complete responses. The workflow we really work through in Leucid is to try and improve each aspect of the process as we move forward. We want to optimize the CAR to function as efficaciously as possible, pair this up with appropriate armoring, and have an optimized and rapid manufacturing process to really get these therapies to the patient as quickly as possible.
These can then either go into an autologous therapy or, of course, into our allogenic cell chassis based on Gamma Delta T cells.
So if we go back to the way CARs are traditionally structured, they form this kind of linear structure whereby the targeting moiety is fused in frame to a co-stimulatory domain and then an activating domain. This is your run-of-the-mill second-generation CAR, which has shown so much efficacy in the hematological malignancy space. When it was clear that this wasn't really going to have sufficient potency to start to truly impact solid tumors, people quite understandably started to bring in an additional co-stimulatory domain, often one that acts synergistically with the co-stimulatory Domain one. The thinking behind this is an endogenous immune response. You get more than one co-stimulatory signal sequentially or even simultaneously, and that improves the efficacy of the overall response. The issue with the third-generation structure is the fact that, by virtue of it being a linear molecule, that second co-stimulatory domain is positioned distally from the plasma membrane, i.e., not where it would naturally be found within a T cell. We've shown quite convincingly that this results in sub-optimal signaling from that second co-stimulatory domain.
Having said all this, it would be remiss of me not to mention the recent results that came out of Locatelli's group in Rome, where they treated patients with high-risk neuroblastoma using a third-generation CAR T-Cell targeting GD2 or ganglioside D2. They achieved an overall response rate upwards of 60%, 33% of which were complete responses. So, what this data clearly shows is that we can put to bed the argument that CAR T-Cell technology does not work in solid tumors. This is clear evidence that it can, but we just need to improve the breadth with which we can get that to function.
The way we've gone about this in Leucid is we've gone back to look at this CAR and said, "Well, I think the idea of providing the additional co-stimulation is correct, but it's been done in a sub-optimal way. How can we improve this? How can we change the structure of the CAR to optimize the signaling through it?" To this, we've developed two separate platforms. The first of which we've termed the parallel CAR or P-CAR. In this, we co-express a second-generation CAR alongside what we call a chimeric co-stimulatory receptor. This second receptor provides just the co-stimulatory signal and allows them to act simultaneously and synergistically. Importantly, it positions that second co-stimulatory domain at the plasma membrane where it can function most optimally.
The beauty of the P-CAR technology is it is entirely plug-and-play. So, you can use the two binders to target the same epitope within the same antigen, they can target different epitopes within the same antigen, or they can target different antigens altogether. And we have shown this quite comprehensively, and I've provided some references there for anyone who wishes to go and look at that further. The reason I provided those references—I'm actually going to focus most of my talk today on our second platform technology, which we've termed an adapter CAR. Again, we would still put this under the lateral CAR umbrella because the signaling domains are laterally spread across the plasma membrane. We're building out, not down. We're not pushing anything away from the plasma membrane. But the adapter CAR structure was based on the fact that we took a step back and we looked at the way Mother Nature builds immune receptors, and all of them use what's termed as a multi-chain immune receptor. The binding domain and the signaling domains are separate proteins that then associate at the plasma membrane, often due to complementary, um, charged residues allowing them to assemble across the plasma membrane. But therefore ensuring that all of the signaling units are nicely up at that plasma membrane where they work most efficiently.
So, with this in mind, Leucid's clinical asset is based on NK G2D. NKG2D is an adapter structure that is found naturally within the body, traditionally within NK cells and CD8 positive T cells, along with Gamma Delta T cells. It targets a family of ligands, and these are stress ligands, i.e., they're upregulated by cells undergoing cellular stress. Tumors are nothing if not stressed, and therefore, the ligands are found on over 80% of tumor types, both solid and hematological malignancies. But they're also found on a number of cells within the tumor microenvironment. This includes cells such as stromal cells, which act as a barrier to CAR T-Cell infiltration but also suppressive immune cells like regulatory T cells and myeloid-derived suppressor cells. Because they are markers of cell stress, they are rarely found on healthy tissue, certainly no other cell surface where they will be able to be targeted by a CAR T cell. So, our asset, New 002, is based on NKG2D itself, allowing us to target these NKG2D ligands, and it mirrors the adapter structure formed naturally by NKG2D. Throughout this presentation, we'll be comparing the function of this against a linear NKG2D CAR known as N Theta.
Now, before we undertook this analysis, we needed to check whether our adapter CAR structure would actually provide the type of efficacy we would expect. Was it able to recognize NKG2D ligands, and was it able to achieve reasonable efficacy with these CAR T cells? So, to do this, we undertook a series of in vitro experiments.
I think it's very important when developing a clinical protocol or what could be considered as a clinical asset to really make sure that your pre-clinical research is incredibly stringent. The first person you have to convince of the efficacy of your potential asset is yourself. Are you truly going to want to put somebody through a clinical trial if you don't really believe that the asset you have developed has the likelihood of achieving some form of response? Probably not. And therefore, the best way of convincing yourself is to make your pre-clinical research incredibly stringent. For this reason, we often use something called re-stimulation assays as an in vitro marker of efficacy.
This is where we will co-culture the T cells and the tumor cells at a defined ratio for a defined time point. Once those T cells have cleared the tumor cells, we will add in fresh tumor cells, and we will continue to do this experiment again and again and again with the same T cells until they fail to kill that tumor. Basically, what we're looking for is functional persistence of those T cells. How many times can they undergo activation and tumor cellulitis before they lose the ability to do that. What you can see here from these graphs is that the LU02, so the NKG2D adapter CAR, was able to undergo significant numbers of re-stimulation on a number of different cell lines. I've shown here mesothelioma and pancreatic ductal carcinoma compared to T cells expressing just wild-type NKG2D or unmodified, as shown by untransduced. Importantly, what we saw was not only were these T cells able to undergo multiple rounds of lysis, but this was followed by quite substantial proliferation of these cells in response to the antigen. What was very interesting is they started to generate this kind of Sawtooth pattern or this expansion-contraction-expansion-proliferation pattern that you would associate with an endogenous immune response.
And what this data, therefore, showed us was these CAR T cells were able to recognize the NKG2D ligands, they were able to respond, lyse tumor, and proliferate in response to those. We then turned to the Omni Pro 12 from Axion to look at the kinetics behind this. A lot of the initial work was done with endpoint assays, which are very important because they show whether your T cells are functional. But you can combine that with kinetic data you get from more regular monitoring, which machines such as the Omni Pro 12 allow you to look at the kinetics behind these re-stimulations.
Here you can see, this is just looking at two rounds of stimulation. At 72 hours, we added some fresh tumor in, so the T cells had managed to lyse most of the tumor. We added fresh tumor in at 72 hours, and you can see that the reduction in tumor viability was much quicker the second time around as a result of the fact that these T cells had nicely proliferated. As you can see from the blue line, non-transduced T cells had no efficacy or no effect upon the tumor cell lysis.
I mentioned earlier that one of the benefits of targeting NKG2D ligands, apart from their lack of expression in healthy tissue and the breadth of expression across a wide range of solid tumors, was the fact that you are able to target other cells within the tumor microenvironment, including stromal cells. Now, this is incredibly important in a number of diseases but probably best shown by pancreatic cancer, where up to 90% of the tumor is not made up of malignant cells but it's actually stromal cells. It's the job of these stromal cells to protect those malignant cells, not only as a structural barrier but they actually produce a number of chemokines that actually tempt the T cells into them, something called the "stroma highway hypothesis". The T cells get attracted to the stromal cells, get stuck there, and therefore never actually make it to the tumor itself. So, what we wanted to look at is if you co-culture the tumor cells and some stromal cells together, are we able to target those malignant cells and potentially the stromal cells as well? Or does the influence of the stromal cells have an effect on the tumor themselves?
We'd actually shown that stromal cells, certainly in vitro, upregulate NKG2D ligands anyway. And you can see from this data here that when the T cells were co-cultured with the tumor alone or with tumor and stroma together, we were still able to get a complete eradication of the target cells.
We then decided to look at this a bit more thoroughly. Were those stromal cells just being lysed as a result of some form of bystander effect, or the T cell is actually able to target them directly? So, we actually utilized a predecessor of the Omni Pro 12, still from Axion, whereby we could do some time-lapse microscopy to look at where our CAR T cells, these NKG2D adapter T cells, went. So, you can see here we've mixed our pancreatic ductal adenocarcinoma cells with some pancreatic stromal cells, and then we've put our NKG2D CAR T cells on top. If we play this video, what you can see is actually really quite fascinating. The CAR T cells go to the stromal cells and lyse those stromal cells before they go after the malignant cells. This very much fits with the stromal highway hypothesis, but the important thing is those T cells are able to recognize and lyse those stromal cells after which they're then able to go after the tumor cells.
This video was taken over the course of the 24-hour time point with a one-to-one effector-to-target ratio of T cells to tumor and stromal cells, so you can see this still gives a very effective therapeutic effect.
We wanted to make this a bit more stringent, so we developed some spheroids, 3D models of the tumor, including the stromal cells. And you can see compared to NKG2D or untransduced T cell controls, neither of which showed any effect upon the spheroid when grown on its own, those T cells co-cultured with the LU02 adapter CAR T cells showed enormous complete eradication of both tumor and stroma.
Again, when we went back to do some time-lapse microscopy, this time on a tumor spheroid, you can see that the LU02 T cells are able to penetrate into the depths of that spheroid really quite rapidly, and it almost causes it to implode. You can see that it causes complete disaggregation of this spheroid, resulting in incomplete spheroid lysis.
Of course, in vitro efficacy is one thing. How do these T cells function in vivo? So here, we took two different tumor models. On the left, you can see a refractory pancreatic cancer model, and actually, the blue dots you can see in that are the T4 that achieved stable disease in head and neck cancer patients in the clinic. Up against our New 002 NKG2D targeted second-gen adapter CAR, shown in green. On the right-hand side, you can see a mesothelioma xenograft. Again, compared to T4, LU02 gives very robust efficacy. The important thing is, again, coming back to the stringency of response, not only do we get very nice tumor regression after T cell infiltration, if we then re-challenge the mice with the same tumor weeks and weeks after that T cell infusion, we get a complete regression or a rejection of that re-challenge, showing that not only are we achieving good efficacy but benefiting from the persistence of these T cells as well.
So we're not the only people to have developed CARs targeting NKG2D. There are ones that have been in the clinic and shown some level of efficacy and, more importantly, safety in the clinic. But these have been based on what we would term a linear CAR architecture, so this traditional linear structure. It was logical for us to then compare our novel lateral adapter CAR architecture versus this kind of gold standard in NKG2D CAR technology, which is the linear structure.
You can see we went back to our re-stimulation assays in vitro, and you can see we see significantly more rounds of re-stimulation and substantially better proliferation when these T cells use this adapter structure that is so prevalent in endogenous immune receptors.
When we went back to our spheroid model again in vitro, you can see here that compared to untransduced T cells, those treated with the linear NKG2D CAR did show some level of anti-tumor efficacy. You can see that while you can still macroscopically see these spheroids in the factor tube, when you then disaggregate them and run them through the flow cytometer, you can see a reduction in tumor and stroma and an increase in the actual T cell number. These are all matched with a number of spiked beads we're actually basing this on the number of beads to make sure that we're counting correctly between them. When you do the same with the LU02 NKG2D adapter CARs, you see a complete eradication of the tumor and stroma. Not only can you not see them macroscopically, but when you actually try to disaggregate what's in the tube and run it through the flow, you really just pick up T cells.
How about in vivo? For this, we developed a patient-derived xenograft model of mesothelioma, so this is actual patient tissue. And you can see that compared to control T cells, the NKG2D T cells, shown in kind of dark purple, which had no increased efficacy over PBS, those CAR T cells with the linear NKG2D CAR also showed negligible anti-tumor efficacy. In contrast, the adapter CAR T cells, shown in green, had a complete response in six out of seven mice with a very good partial response in the final mouse, and again, this was then durable well past 200 days. And this led to a substantial improvement in the survival of these mice. However, it's not just the efficacy that you can achieve from these adapter CAR T cells; it's also the persistence. In this model of peritoneal ovarian cancer, you can see that actually compared to the controls (either untransduced T cell or just saline PBS), both the linear CAR shown in pink and the lateral CAR T cell showed very good anti-tumor efficacy. But, like I say, we like to make things very tricky with our pre-clinical research.
So, when we then re-challenge the mice with a fresh bolus of tumor, all the mice that had been previously treated with the linear NKG2D CAR T cells showed a complete failure to control this fresh bolus of tumor, whereas those that had been pre-treated with the LU02 T cells were able to show complete eradication of the tumor. What's important when you see that second vertical line is you can see an uptick in tumor. That's because we're showing that the tumor has taken in these mice. This is done the day after inoculate re-challenge to show that you've actually successfully injected the tumor in, but then that tumor is completely eradicated, showing that those New 002 T cells persist over time.
And so, if we take this out to its extreme, we've now done multiple in vivo models, and this is a combined Kaplan-Meier graph. Essentially, by simply changing the architecture of your CAR, the T cells have gone from simply 7 percent up to 63 percent of the mice still alive at the end when we usually colonize due to advanced age.
So, having improved the efficacy of the CAR simply by changing the architecture, we are at a point where we feel we've optimized that CAR as well as we can, and we now wish to pair that with appropriate armoring technology to really further improve the efficacy of our CAR T-cell product.
And it's areas like this, things that machines such as the Omni Pro 12, and in this case, the Omni Pro 12 that can shuffle through 12 plates automatically and provides us with fluorescent imaging, as well, can be very useful. A lot of what I've shown has been endpoint analysis, but understanding the kinetics that happen within that is also very interesting.
Here we'd undertaken some armoring strategies, and I'll play both videos simultaneously. The one on the left is non-armored; the one on the right is armored with something we thought would work, but actually, you can see it had the opposite effect; it impacted the ability of these cells to work as efficiently. Now, in a case that this is kind of black and white as this, we would have been able to tell the difference in these constructs through our standard endpoint analysis. But in others where we've been comparing two CARs, you can see, again, here we're using the Omni Pro 12. We get the videos that go with it, which are very informative because they show us where the T cells are going, what tumor type they're going to first if we do co-cultures, but also, you know, if we've done endpoint analysis here, we would have concluded that these two CARs function almost identically. But using the kinetic analysis, you can show that one functions much more quickly than the other, reaches its plateau much more quickly. So this kind of information makes the analysis and also the design of your next-generation constructs much more powerful because it's much more informed with what I would say is that what we went on to do is arm LU02, our adapter NKG2D CAR, with a homing receptor to become what is our clinical asset known as Blue 011. The idea here is if we go back to those hurdles associated with CAR T cell therapy for solid tumors was around the idea of getting those T cells to the tumor more efficiently.
When we went to our pancreatic cancer model, this is subcutaneous pancreatic cancer with the T cells administered intravenously, you can see that the linear NKG2D CAR did have some level of anti-tumor efficacy compared to controls. This was further improved by that switch from a linear architecture to a lateral architecture, so NZ to LU02. But by then including a homing receptor to our armored version of the adapter CAR, you've got a complete response in all mice that lasted well out to nearly 180 days.
What we also did is harvest some of those tumors from a subgroup of mice early on in the experiment to do some immunohistochemistry on them to look for T cell infiltration based on CD3 staining. You can see that Blue 011 T cells infiltrate much more efficiently into these subcutaneous tumors compared to the non-armored version. In addition to improving the efficacy, this potentially also allows us to reduce the dose we need to give to achieve that efficacy. Now, that's important not just from reducing the burden on your manufacturing process, and while we're very happy with the robustness of our manufacturing process, if you can get away with having to manufacture less, that's always a benefit. But also, it reduces the potential toxic effects that high doses would have in patients.
So, we went back to our pancreatic cancer model, and you can see here that we saw no real response with the linear CAR. But at the same dose with the lateral CAR, be it armored in red or unarmored in green, we saw a complete response in all mice. When we reduced down the dose two and a half-fold, the non-armored adapter CAR started to show still very good efficacy but relapsing in some of those mice, whereas the armored version of the CAR at the lower dose level continued to give a complete response in all mice outwards to nearly 200 days, and that resulted in a significant improvement in the survival of these mice's ability of equivalent efficacy but at a lower dose.
Again, if we now take this to its final conclusion, by armoring your T-cells effectively, you get a further uptick in efficacy. So, in essence, what we're achieving is by optimizing the structure of the CAR, what we term the adapter effect, you get a massive increase in efficacy to improve the baseline efficiency of your CAR T-cells. This can then be paired with the appropriate armoring technology to further increase the efficacy of your therapy. And what this has resulted in is the fact that mice treated with our armored version of our adapter CAR have an 84% chance of survival at the end of the experiment compared with just 7% of mice treated with a linear NKG2D CAR.
So with that, I'd like to finish and thank you for your attention. I hope you found it useful. What I'm hoping to have been able to convince you of today is the fact that optimization of CAR structure, which has been an understudied area in the field, can provide real benefit. And this is through juxta-membrane positioning of the natural signaling domains, which results in improved anti-tumor efficacy, proliferation, T-cell persistence, and cytokine secretion. By doing this, you can then build in additional armoring technologies to create truly transformative therapies.
Importantly, the baseline switch of your CAR structure requires very little additional engineering, if any, compared to that you would do for your standard second-generation or third-generation CAR T cells. So, in real estate parlance, it's all about location, location. With that, I'd like to finish and also just acknowledge the team, the incredible team of scientists we have at Leucid who've developed a lot of these technologies and undertaken experiments to show them. Also, the people involved in the trial that I showed right at the start, most notably the T4 patients and their families. Thank you very much.
Zhong Yu: Well, thank you very much, Marc and Stacie, for your excellent presentations. We will now start the Q&A portion of the webinar. If you have a question you'd like to ask, please do so now. We'll answer as many of your questions as we have time for.
Okay, let's get started with our first question, and that is for Marc.
Marc, this question is from Victoria: "What do you think is the reason for the CAR T cells attacking the stroma cells first? Do they express higher levels of NKG2D?"
Marc Davies: Yeah, that's an excellent question. Thank you, Victoria, and thanks, everyone, as well. Um, they don't express high levels of the ligands. Um, they do secrete an awful lot of kind of key um, T-cell attractants, so certain chemokines that T cells are specifically attracted to. As I mentioned, it obviously is a hypothesis within the field, this so-called stromal Highway hypothesis, but the idea is that the T-cell the tumor cells, sorry, but basically educate the stromal cells to firstly act as a barrier and secondly act as a decoy. So they want to tempt the T cells into the stromal cells so they never actually make it to the malignant cells. So we think it is that chemokine gradient down, which causes the T cells to go to the stroma first. As I mentioned in the presentation, the benefit of the fact that the stroma cells express the NKG2D ligands is we can actually target those as well. I guess that's an additional benefit you have there.
Zhong Yu: I have a question actually from your time, also addressed to Marc. "Do you see similar trends targeting different antigens than NKG2D?"
Marc Davies: Yeah, so I adapt the CAR technology is centered around NKG2D. With our parallel CAR or PCAR technology, which I kind of touched on in the presentation but didn't really show much data because we've published quite a bit on that. We've done multiple targets, and I suppose the benefit you have there is, like I said, because you have two different binders, you can go after either the same antigen or different antigens. And we've done that across a number of targets, um, CSF1R, CD19, um, HER2, and really showing the kind of flexibility of that. And the benefit of the PCAR has over second generation is very similar. It improves T-cell persistence, their ability to continue to secrete cytokine through multiple rounds of stimulation, and what we call also functional persistence. They don't exhaust quickly. They're able to undergo more rounds of tumor cell lysis and proliferation. So it is very, it does seem to be very much down to the architecture of these, um, and the optimal signaling you achieve through those.
Zhong Yu: Got it, thank you. I have a question from Guido, and perhaps Stacie would you min danswering this question unless Marc knows balance as well. "How long does it take to image one full plate with the Omni?" I guess that's a 96-well plate. Then in brightfield alone versus bright field plus fluorescence.
Stacie Chvatal: Sure, so thanks. I'm happy to answer that one. In brightfield alone, you can do the whole plate brightfield scan. It takes around nine minutes and then a couple of minutes for processing and fluorescence. It really varies depending on the number of snapshots that you're taking per well and the well plate that you have, but it can range anywhere from just a few minutes up to maybe about 20 minutes or so, depending on your experimental setup.
Okay, I hope that answers your question. Feel free to contact us too if you have more specific questions on the times.
Zhong Yu: A question for you, Marc, from Kodeep. "In your models, do the mice treated with armored cars survive longer due to the suppression of GVHD versus more potent T-cell killing, or do your engineered cells induce GVHD in the mouse models?"
Marc Davies: Yeah, it's a fantastic question, obviously something that we've been a bit mindful of because we do tend to achieve such good tumor eradication in these models that the experiments go out you know 200 plus days. We've not seen any evidence of GVHD. Standardly, that would be more, um, you know, we're obviously giving a purified T-cell product, um, you'd expect GVHD a bit more where you can have than APCs that cross-react and provide uh Xeno-reactive peptides. But nonetheless, obviously, it's something we've been mindful of, but we've not really seen anything. One of the benefits of the Homing receptor is it also should pull the T cells directly into the tumor, but obviously we then do eradicate that tumor, and so it is interesting the fact that we haven't then seen also, obviously, subsequent Xeno-reactivity. But I think, you know, the next steps really are to do the T-cell Imaging as well. We've obviously done some level of T-cell Imaging in the mice, but what we think is happening is obviously we get massive expansion, eradication of the tumor, and then almost a complete contraction of that cellular population which sits at very low levels. We get some level of engraftment in those mice, and then when we re-challenge, we get a re-emergence of those clones. So it's actually quite a good model for what happens also in the human body, I guess, then as well as a mouse model can be. Yeah, yeah, yes, obviously.
Zhong Yu: I have another question for you, Marc. "Thanks for the nice presentation. Do you think that the lateral strategy for CAR T cells is better than the linear structure for all types of CAR?"
Marc Davies: Issue, but the structure simply isn't optimal. We've done a lot of work traditionally in third gen, and people have their own preferences for costim, but it would normally be CD28 followed by CD4 or BB. Data actually that was generated by RCSo John Maher, right back in the early 2000s when he started working on this, has already shown that you cannot put CD28 away from the membrane. So he made second-generation cars as 28 Zeta or Zeta 28, and the 28 when it's downstream of the CD3 Zeta, it simply doesn't work at all. In a third-generation car, like I say, they tend to be 28 4 MBB Zeta, and we've done quite a bit of work looking at using NF Kappa B as a reporter for form BB signaling and basically shown in the third-generation structure, the NF Kappa B signaling you get from that form BB is significantly less optimal as if it were you just using a 4B second-generation car. So clearly, that form BB is signaling sub-optimally. If you then put them into a PCAR structure where that 4B is back up at the membrane, and CD28 is both off the membrane because you're obviously expressing them off two separate receptors, you get that better, more optimal signaling. In fact, the level of NF Kappa B signaling you get through the form BB is slightly improved on even a second gen. And so we have tested it fairly exhaustively, and we would suggest that this improved structure should work across all types of cars. But, you know, we're willing to give it a go if people have someone they want us to test. We're willing to put different people's individual binders into the PCAR platform and prove to them that it does work for their CAR as well.
Zhong Yu: That's awesome. You guys will know how to reach out to Marc and get the collaboration started. I think I have time for one last question, and I think this is perhaps better for you, Stacie: "The microscope looks really interesting. Can we test this in our lab as well?"
Stacie Chvatal: Yes, absolutely. We have account managers located in multiple places across the world, and they'd be happy to bring in an Omni BR and Omni FL to your lab to test in a demo. So certainly, don't hesitate to reach out if that's something you're interested in.
Zhong Yu: Okay, well, thank you again, Dr. Davies and Dr. Chvatal, for your time today and your important research. Before we go, I'd like to thank the audience for joining us today and for the interesting questions. We had not time to answer them all, but for those that have submitted during the on-demand period and that we haven't been able to answer, we will address them by the speaker via the contact information that you left behind at the time of registration.
This webcast can be viewed on demand by LabRoots, and LabRoots will alert you via email when it is available for replay. We encourage you to share that email with your colleagues who may have missed today's live event. Until next time, goodbye!