How do we get closer to perfection? It starts with looking at the complete picture
By Jim Ross, CTO and co-founder of Axion Biosystems, Atlanta, USA
Published on The Medicine Maker
CAR T-cells shifted the cancer treatment paradigm as soon as the first therapy was approved in the US in 2017 (1). In the decades before CAR T-cell therapy, whether through surgery, radiation, or systemic treatments, such as hormonal therapy and chemotherapy, physicians tried to do what the immune system wasn’t doing on its own: obliterate cancerous tissue through intense intervention. These treatments, however, are risky and exhibit widely variable success rates, leaving the door open to much-needed advancements in cancer therapy.
CAR T-cell therapy brings personalization into cancer treatment to help increase the chance of therapeutic success. For those unfamiliar with CAR T, physicians first remove T-cells from the patient, then scientists genetically engineer those cells to express the CAR protein on their surface – the CAR protein enables them to recognize cancer-specific antigens. Finally, the CAR T-cells are infused back into the same patient, where they stimulate the immune system to attack cancer cells. In effect, CAR T-cells augment the immune system, prompting it to identify tumor cells as foreign objects that must be killed. In a phase II clinical trial, Yescarta proved 92 percent effective in patients with relapsed or refractory indolent non-Hodgkin lymphoma (2).
According to one forecast, the CAR T-cell market is expected to grow to $6.1 billion by 2030 (3). However, developing these potentially life-saving therapies is not a straightforward process. To continue their growth in the long term, CAR T-cell manufacturers must implement rigorous monitoring tools to guarantee their safety and efficacy.
Complex product, complex production
As CAR T-cells therapies become increasingly commonplace, scientists are learning more about the unique challenges involved in their development and manufacture at scale (4). Cell immunotherapy development involves working with living cells that operate via molecular mechanisms that researchers are still working to fully understand. As a result, cell immunotherapy development is inherently more complex than its small molecule or even traditional biologic development cousins. Developers must not only identify the appropriate molecular targets, but also understand the pathways that determine their function. Optimizing the manufacturing process is also essential to produce these therapies as quickly and efficiently as possible.
Given the variability of immune cell activity, scientists must consider several factors as they design cell immunotherapies for patients. One crucial step in creating safe and effective cell immunotherapies is identifying sufficiently specific target molecules. Aimed at the right antigen, a CAR T-cell will attack tumor cells without harming healthy cells, but finding targets that confer both efficacy and specificity to CAR T-cells can be a serious challenge. For example, the most commonly targeted CAR T-cell antigen (CD19) is not present on all cancer cells. Conversely, solid tumors often express multiple antigens that may be present in low levels on the surfaces of healthy cells (5). Beyond identifying cancer antigens, CAR T-cell manufacturers must also establish optimal conditions for the efficient transduction of the CAR gene, for cell expansion, and for cryopreservation. Finally, they must continually assess how all of these factors affect the potency of the therapy.
Cell therapy companies have many molecular targets, cell sources, and bioprocesses to test, making it difficult to identify a standard protocol that will yield a maximally effective CAR T-cell therapy. Therefore, scientists must not only develop in vitro cancer models that accurately reflect the biology of a patients’ tumor cells, but also adopt a cytotoxicity assay that offers a comprehensive picture of how CAR T-cells function in this environment. In vitro potency assays can produce data that indicate how variations in CAR T-cell development protocols impact the cells’ function and can help CAR T-cell developers optimize their workflows during early-stage clinical trials. Furthermore, these assays can enable companies to rank candidate therapies born from different protocols and screen out suboptimal batches, helping to maximize the production of therapies more likely to succeed in treating patients.
Immune cell-mediated killing of cancer cells is a highly dynamic, variable process; the timing of immune cell activity can differ between donors and between batches. Yet, the most commonly used cytotoxicity assays today do not provide the kind of temporal data that cell immunotherapy manufacturers need to assess their products’ behavior in an in vitro cancer model. Common techniques, such as chromium release assays and other colorimetric assays, only capture data at predefined time points, which can lead a developer to miss critical information. The only way to guarantee capture of all the data needed to characterize immune cells is to monitor their killing behavior in real time.
Getting the complete picture
One tool that can provide this real-time measurement of immune cell activity is a bioelectronic assay that employs biosensing electrodes to detect tumor cell viability. This kind of assay measures electrical impedance caused by the cells’ obstruction of current flow between electrodes. Electrodes can be embedded into each well of a microtiter plate, enabling researchers to capture data from multiple cultures non-invasively and in real-time. As living cells attach themselves to the well, impedance rises; as cells die and detach, impedance decreases. The experiment can be replicated in multiple wells, enabling developers to test how variations in dosing and other factors impact CAR T-cell function.
Recently, researchers used a bioelectronic assay to identify a novel CAR T-cell target for glioblastoma therapy by monitoring CAR T-cell-mediated killing over the course of several days (6). Lohitash Karumbaiah and his team first cultured human glioma cells in a 96-well plate containing bioelectrodes. Two days later, they added CAR T-cells targeting GD2 – a common tumor-associated antigen present on glioblastoma cells – to multiple wells at different effector-to-target ratios. They used the bioelectrodes to monitor CAR T-cell-mediated killing of the glioma cells over the course of seven days.
Even at the lowest concentration of 0.1:1, the bioelectronic assay revealed that GD2-targeted CAR T-cells killed at least 50 percent of their target cells, suggesting that GD2 could serve as a potential target in glioblastoma therapy. The researchers also observed reduced efficacy over time in the assay and, using flow cytometry, confirmed the presence of early markers of exhaustion.
The inherent complexity of CAR-T development creates a need for a new level of analytical testing to ensure safety and efficacy of cells. Unlike endpoint assays, bioelectronic assays can capture the dynamics of CAR T-cell activity and provide developers with the flexibility to identify the cells, targets, and protocols that will yield CAR T-cells that are more likely to succeed in patients.
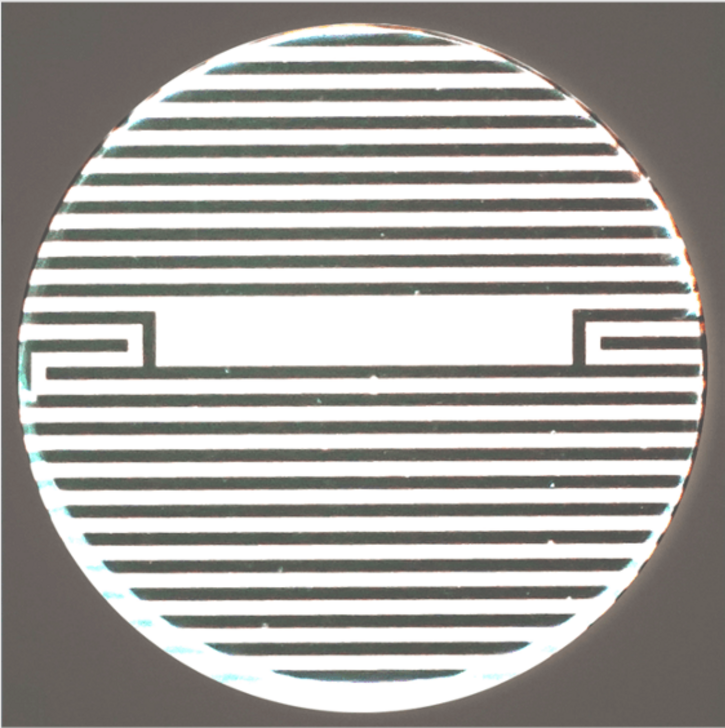
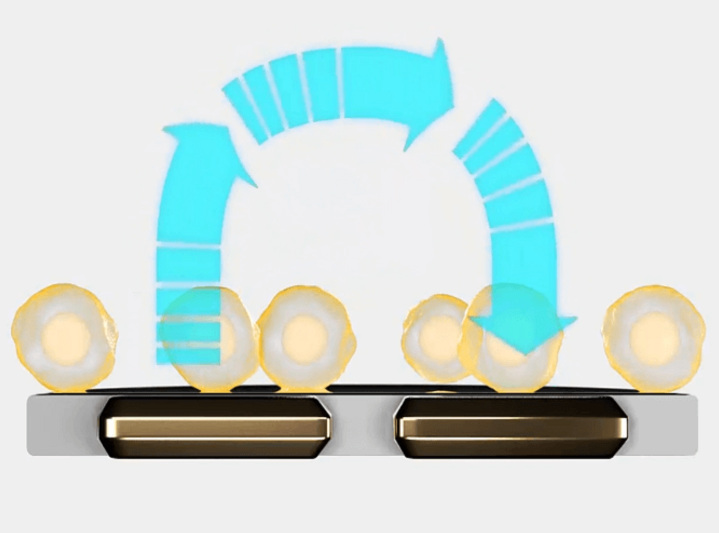
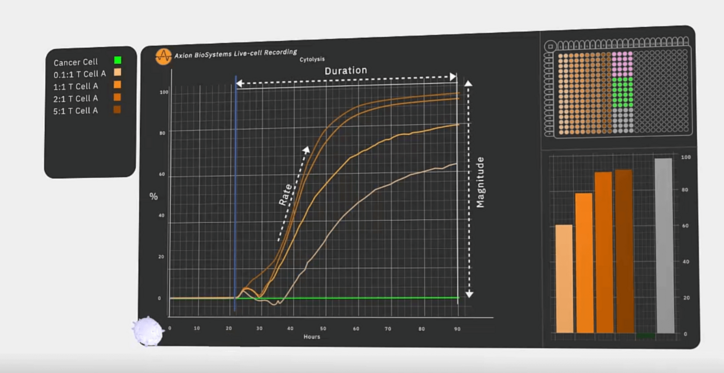
References
- FDA, “FDA approval brings first gene therapy to the United States” (2017). Available at :https://bit.ly/2UWeOWO
- Businesswire, “Yescarta Is First CAR T-cell Therapy to Demonstrate High Response Rates and Durable Clinical Benefit in a Pivotal Indolent Non-Hodgkin Lymphoma Study” (2020). Available at: https://bwnews.pr/3l8cbfc
- PR Newswire, “Global CAR-T Therapy Market Report 2020: Market is Expected to Stabilize and Reach $3,150 Million in 2025 - COVID-19 Impact and Recovery Forecast to 2030” (2021). Available at: https://prn.to/3BLGpdR
- Kymriah, “Find a KYMRIAH Treatment Center” (2021). Available at: https://bit.ly/3i8E05w
- M Martinez and E K Moon, “CAR T Cells for Solid Tumors: New Strategies for Finding, Infiltrating, and Surviving in the Tumor Microenvironment”, Front Immunol (2019). DOI: 10.3389/fimmu.2019.00128
- S A Chvatal et al., “Abstract 2187: Kinetics and potency of T Cell-mediated cytolysis of glioblastoma”, Cancer Research (2020). DOI: 10.1158/1538-7445.AM2020-2187
About the Author
Jim Ross is CTO and co-founder of Axion Biosystems