Cardiac LEAP
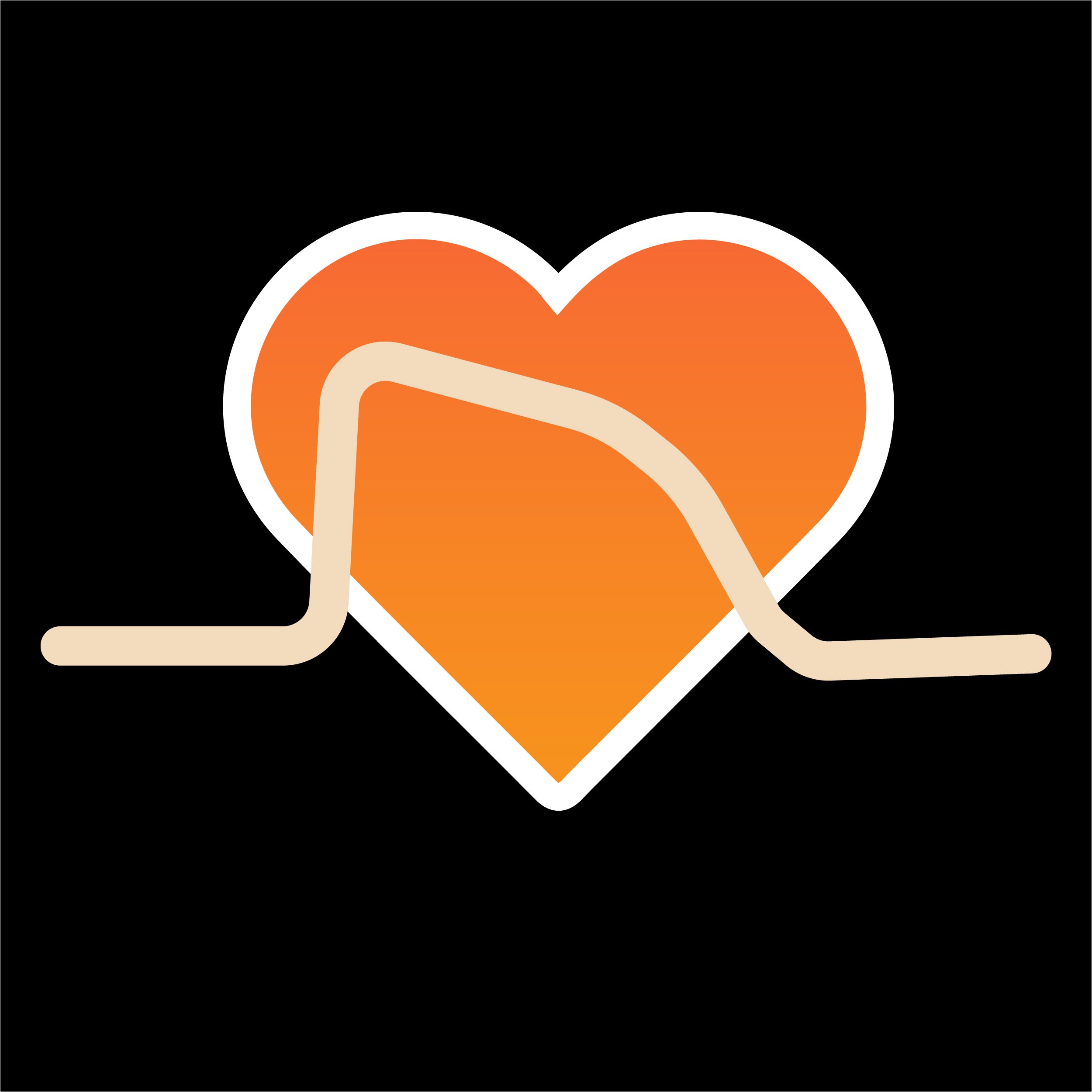
LEAP: For cardiac action potential recordings
The cardiac action potential is an electrical signal characterized by the depolarization across the cell membrane of cardiomyocytes, resulting in contraction of the heart. The shapes of an action potential provide vital information about the cardiomyocyte biology, health, and response to a drug. However, measuring the cardiac action potential traditionally requires invasive techniques, such as manual patch clamp, or labels, such as voltage-sensitive dyes.
LEAP technology enables non-invasive, label-free monitoring of the cardiac action potential in a high-throughput real-time format. LEAP can be used for quantification of action potential morphology, repolarization irregularities such early after depolarizations (EADs), and arrhythmic risk factors such as triangulation. Key metrics, such as rise time, action potential duration (APD), triangulation ratio, and percentage of beats with EADs are all automatically detected by LEAP.
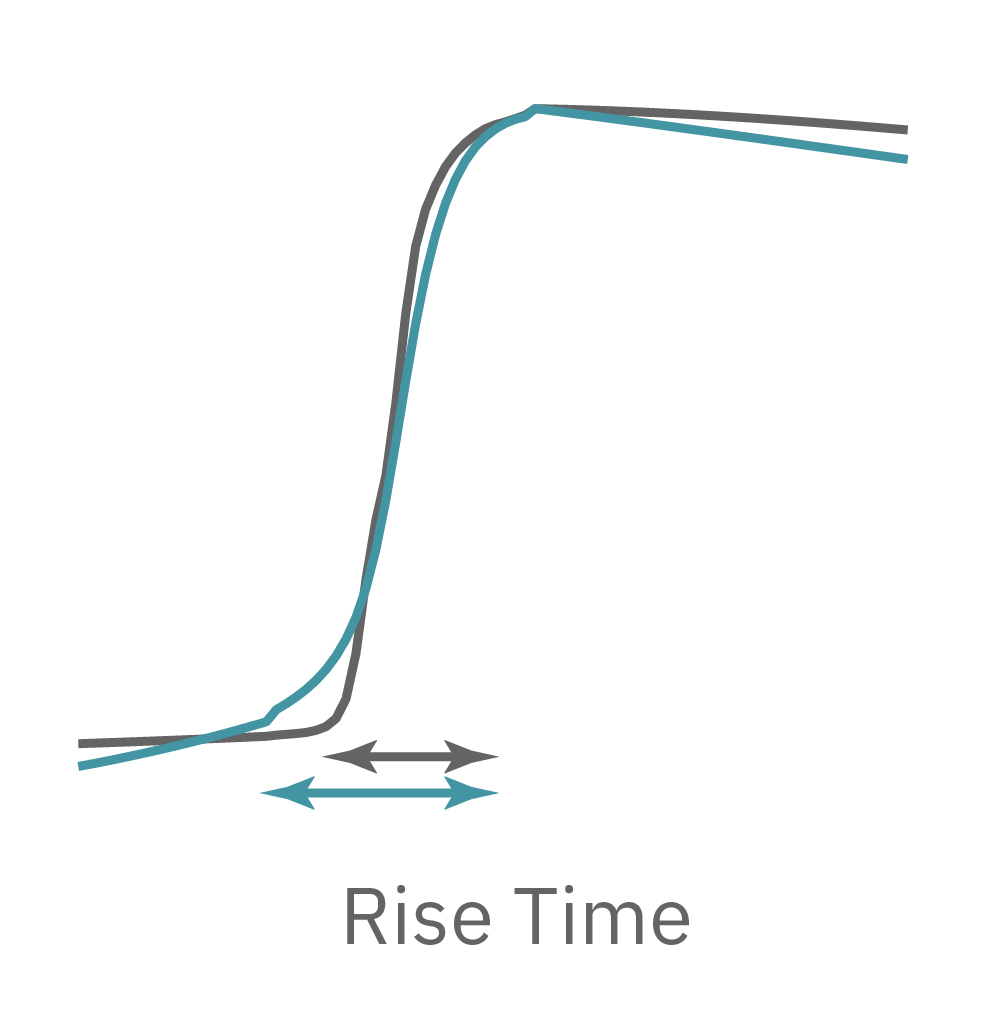
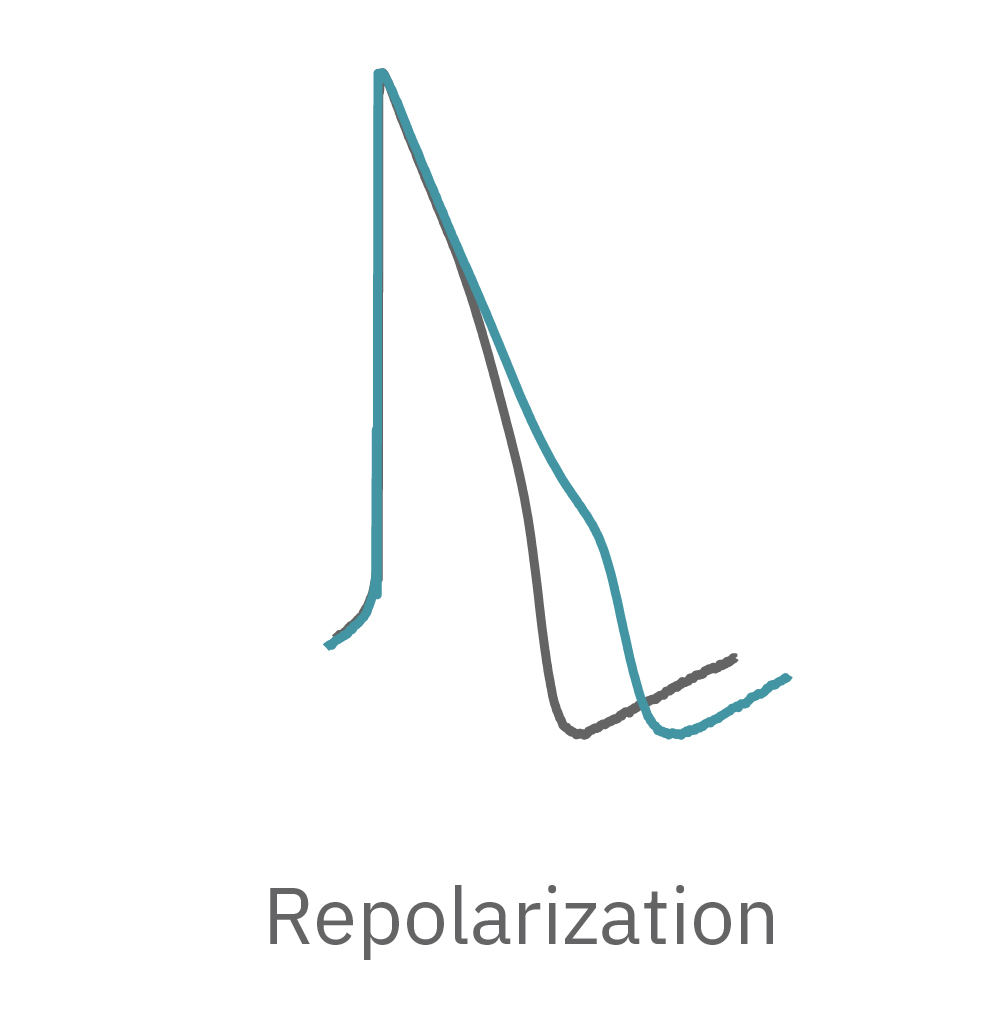
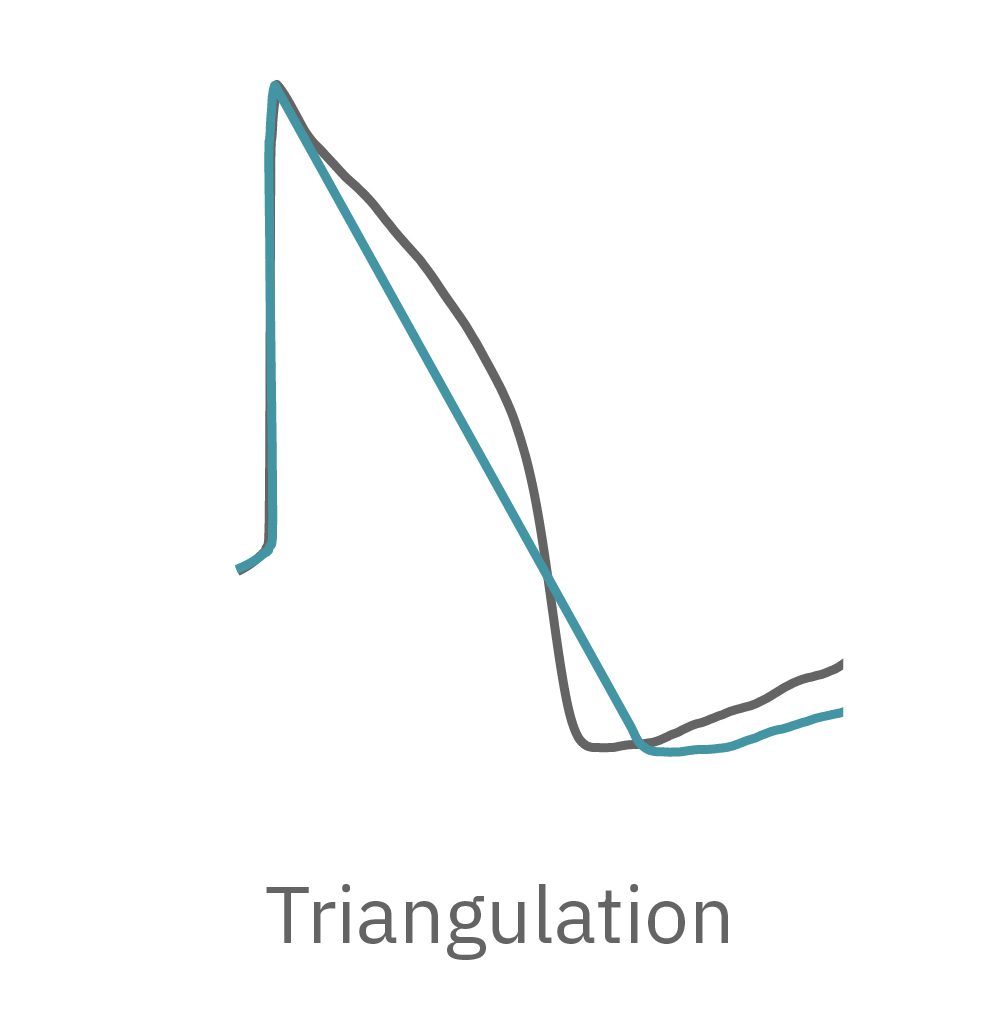
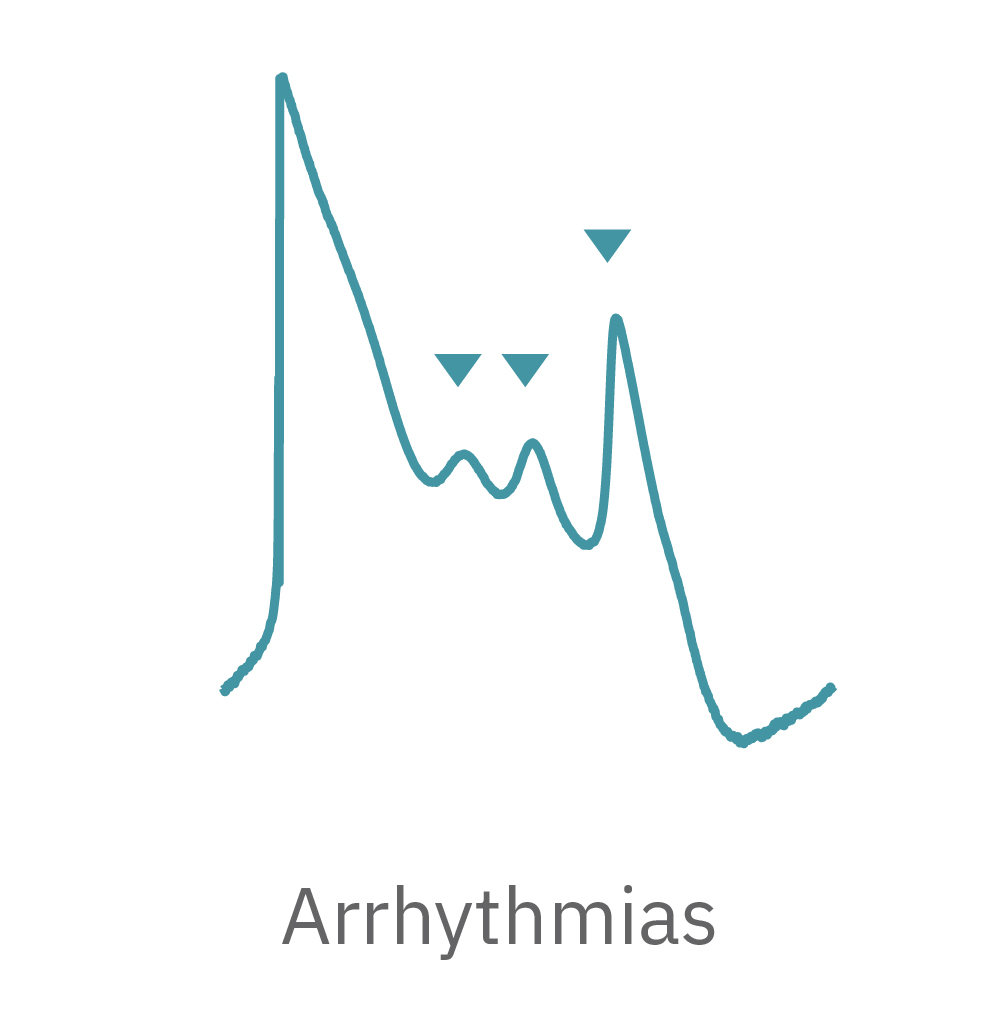
How does LEAP work?
LEAP stands for local extracellular action potential. The theory behind LEAP is similar to that of patch clamp, where the recorded signal amplitude is proportional to the sealing resistance between the electrode and the cell.
In contrast to a field potential signal, LEAP induction increases the coupling between the cells and electrode, enabling the measurement of a much larger action potential signal. The increased cell-electrode coupling is stable for 10-20 minutes or longer, allowing extracellular monitoring of the cardiac action potential without disrupting the underlying biology with dyes or invasive electrodes.
"The LEAP assay addresses an important gap in the field, namely providing a non-invasive solution in recording high quality action potentials from cardiac cells using a high throughput format. The LEAP assay may be a game changer."
Bernard Fermini, PH.D.
Chief Scientific Officer, Coyne Scientific
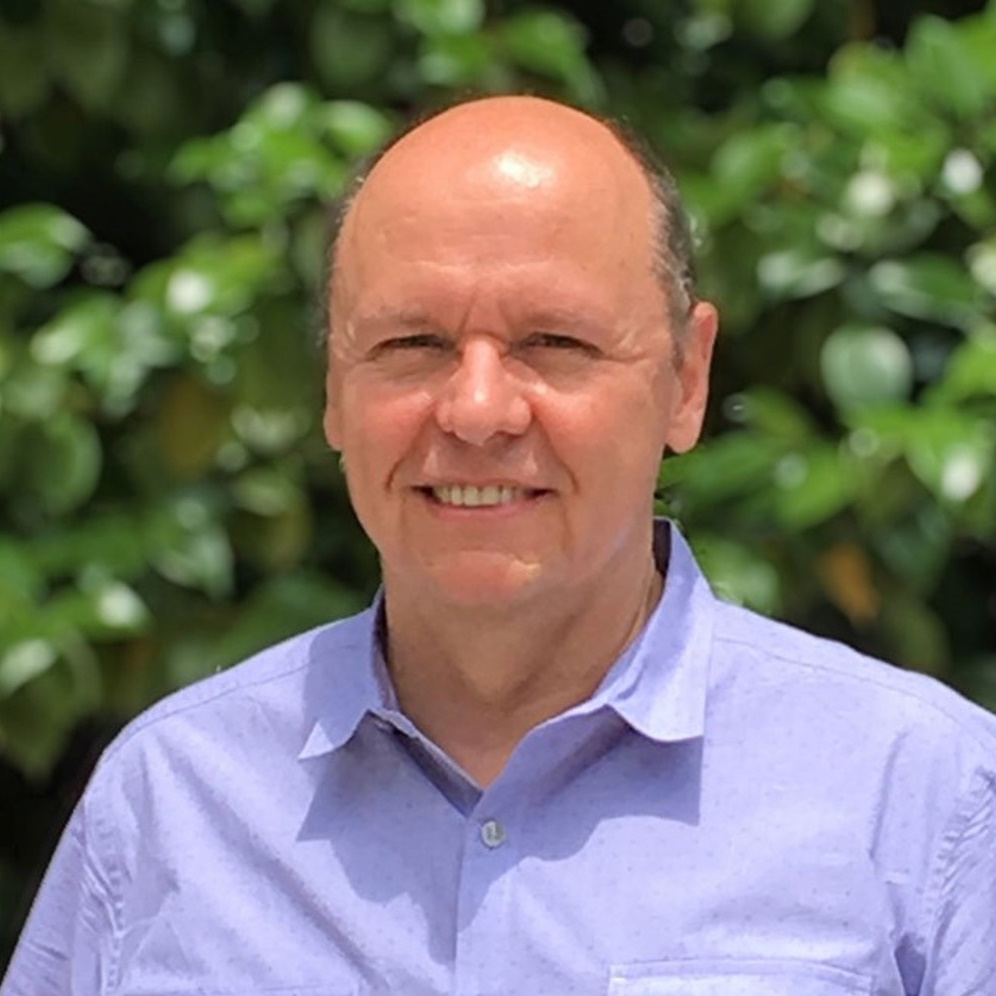
How does LEAP differ from the classic field potential?
The field potential has long been the standard for high-throughput in vitro cardiac electrophysiology. The field potential derives from the underlying cardiac action potential, but more closely resembles the low amplitude clinical electrocardiogram (ECG) signal. The initial depolarization phase is seen as a sharp spike, similar to the QRS complex, followed by the slow repolarization analogous to the T wave. Although the field potential has proven highly effective for high-throughput drug screening and other applications, the field potential shape can obscure complex repolarization irregularities and limit comparisons to gold standard manual patch clamp recordings.
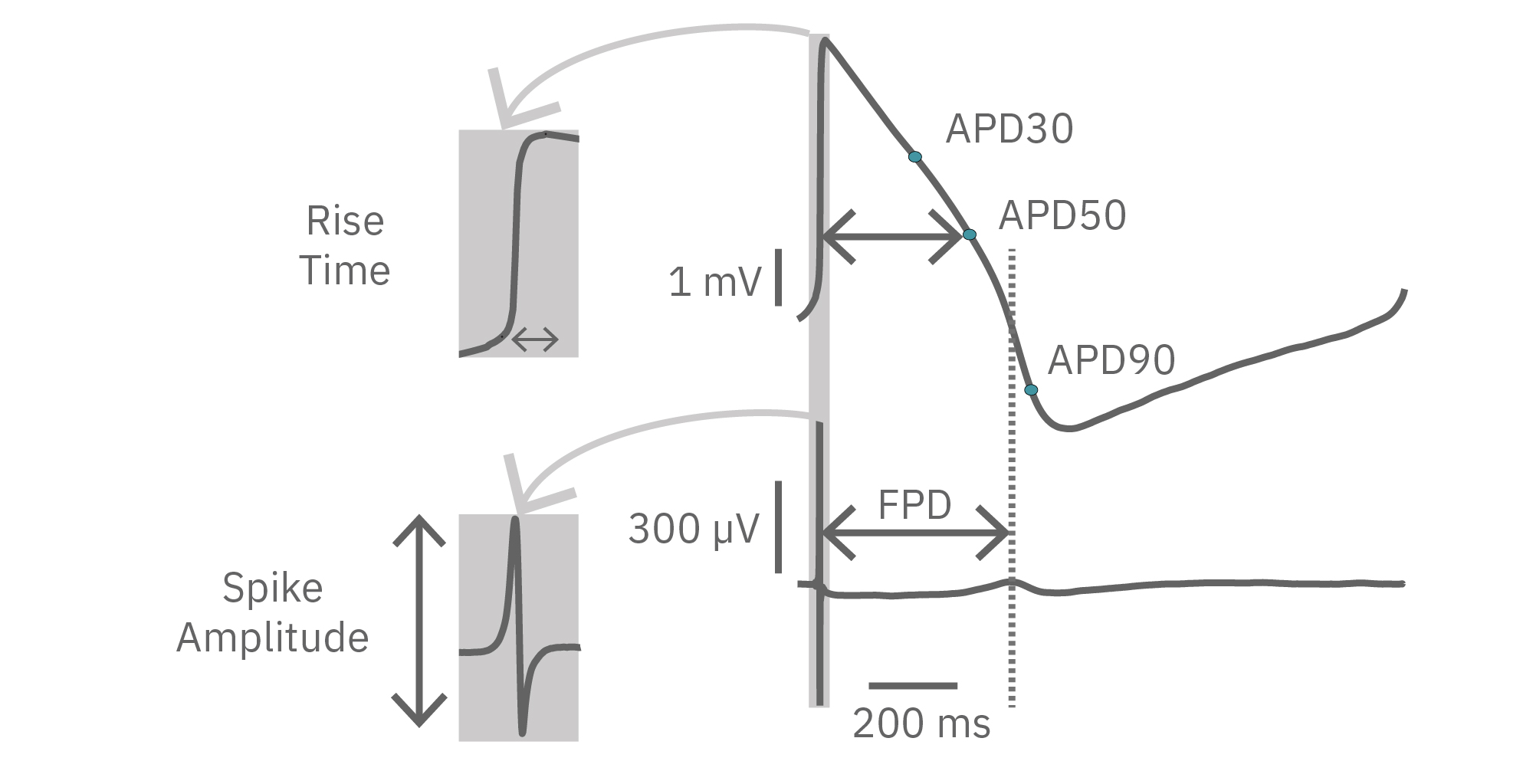
The LEAP signal accurately reflects the shape and duration of the underlying action potential. The large signal allows for automated detection and classification of arrhythmic events, such as notched EADs, rolling EADs, or ectopic beats. LEAP also provides metrics not available from the field potential, such as rise time and triangulation.
Because LEAP operates on each electrode independently, field potential and LEAP signals can be recorded from the same well simultaneously, providing a direct mapping between features of the field potential and the action potential. In this way, LEAP enables confirmation and automation of feature detection.
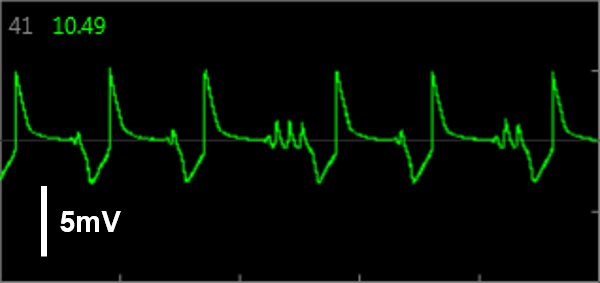
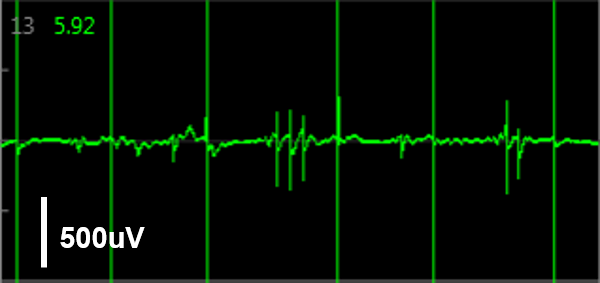
Simultaneous LEAP and field potential (FP) measurements establish translation between FP and LEAP signals. LEAP was induced on half of the microelectrodes in each well of a MEA 48-well plate of iCell CM2. Example LEAP and FP signals from the same well when dosed with E-4031. Depolarization aligned between the LEAP and FP signals, but EADs could be more reliably detected in the LEAP trace.
Advantages of LEAP
Due to the larger features of the signal, the LEAP assay is robust against pharmacological manipulations that can render field potential features difficult to detect.
For example, sodium channel blockers cause a detectable change in spike amplitude. At higher doses though, the spike amplitude can become too small to detect. However, rise time prolongation in the LEAP signal can still be easily measured even at high doses. Similar effects can be seen at high doses of hERG blockers when hERG block begins to impact the resting membrane potential.
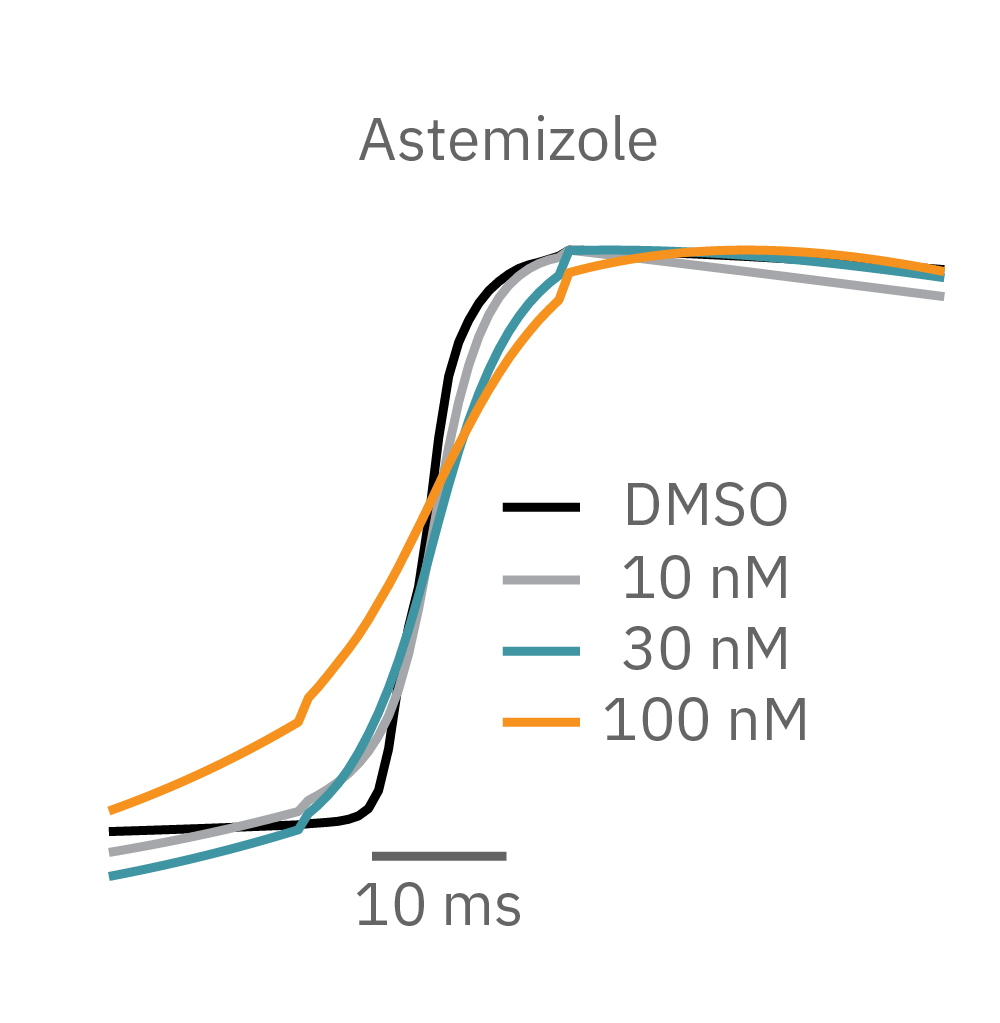
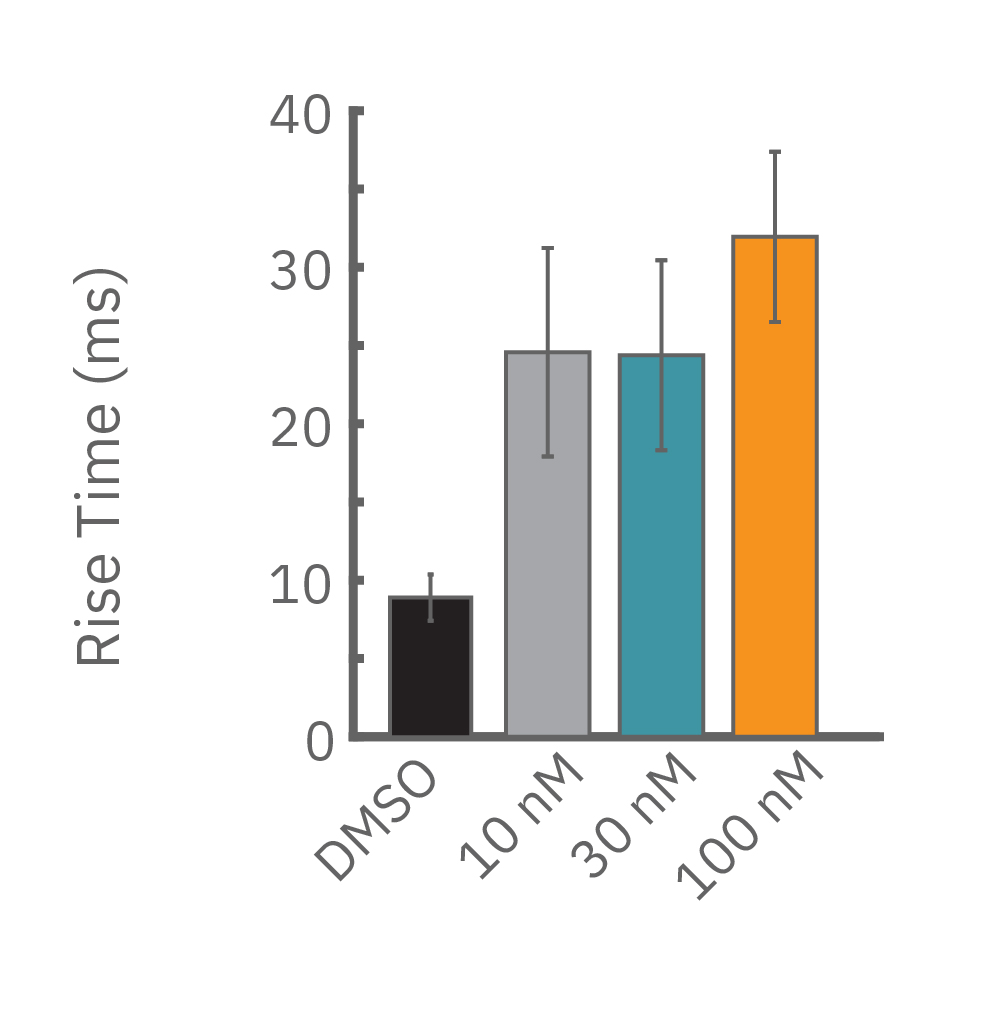
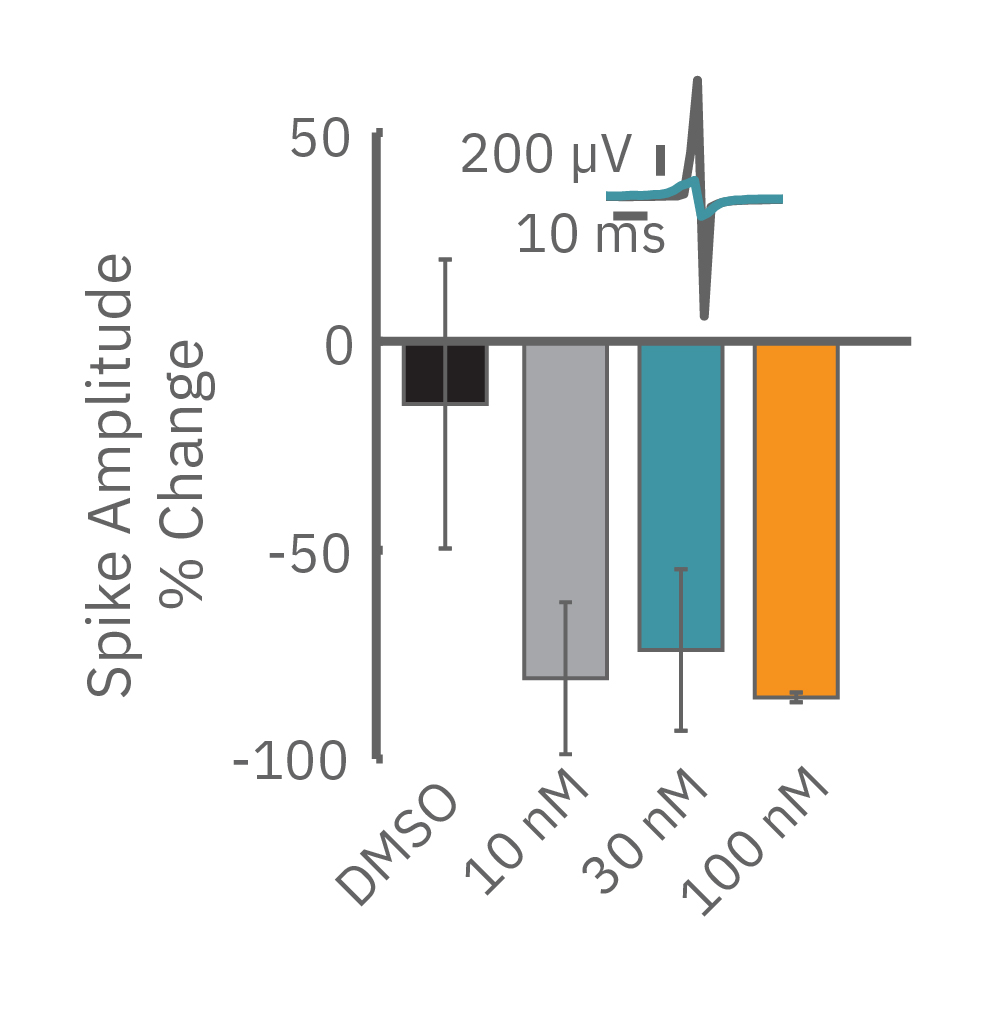
Similarly, compounds, such as terodiline, that induce triangulation can flatten the field potential repolarization feature, referred to as the “T wave”. The resulting broad, small amplitude T wave is difficult to detect and quantify. In contrast, triangulation is readily detectable and quantifiable in the LEAP signal.
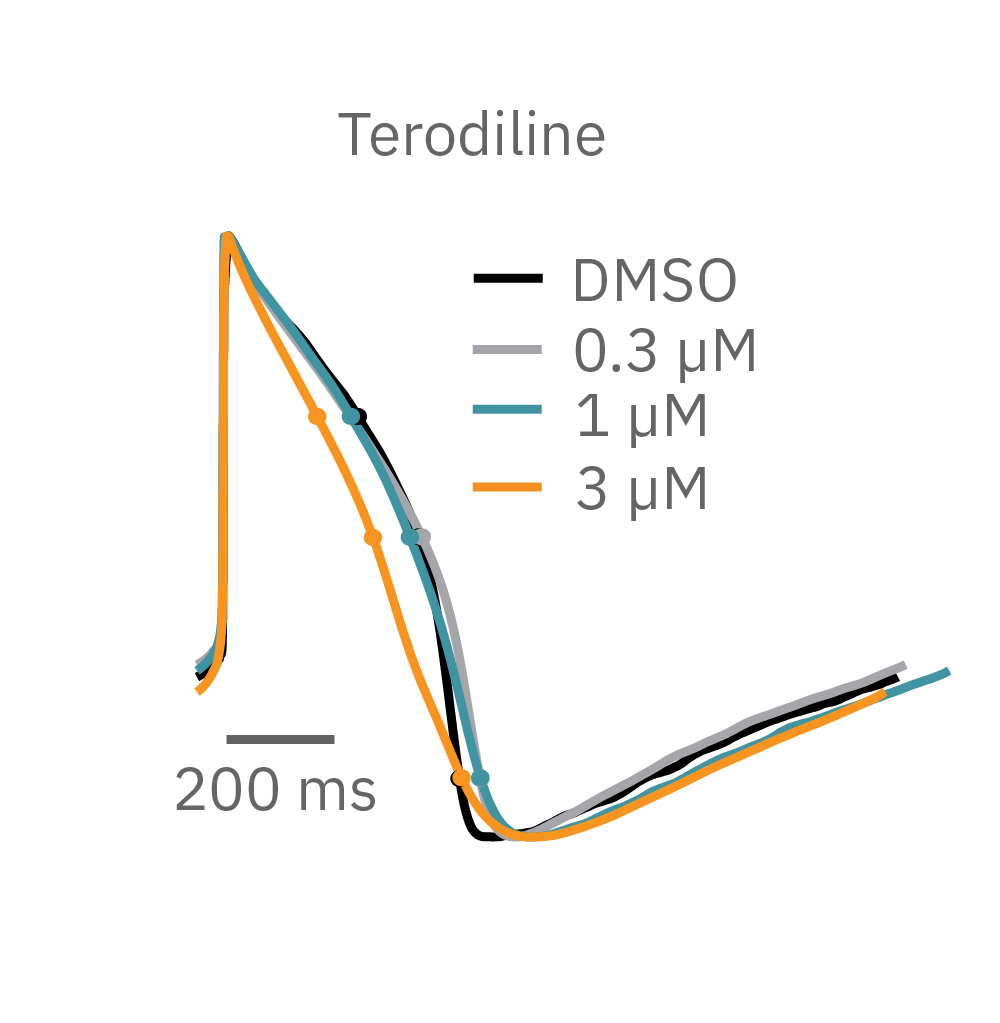
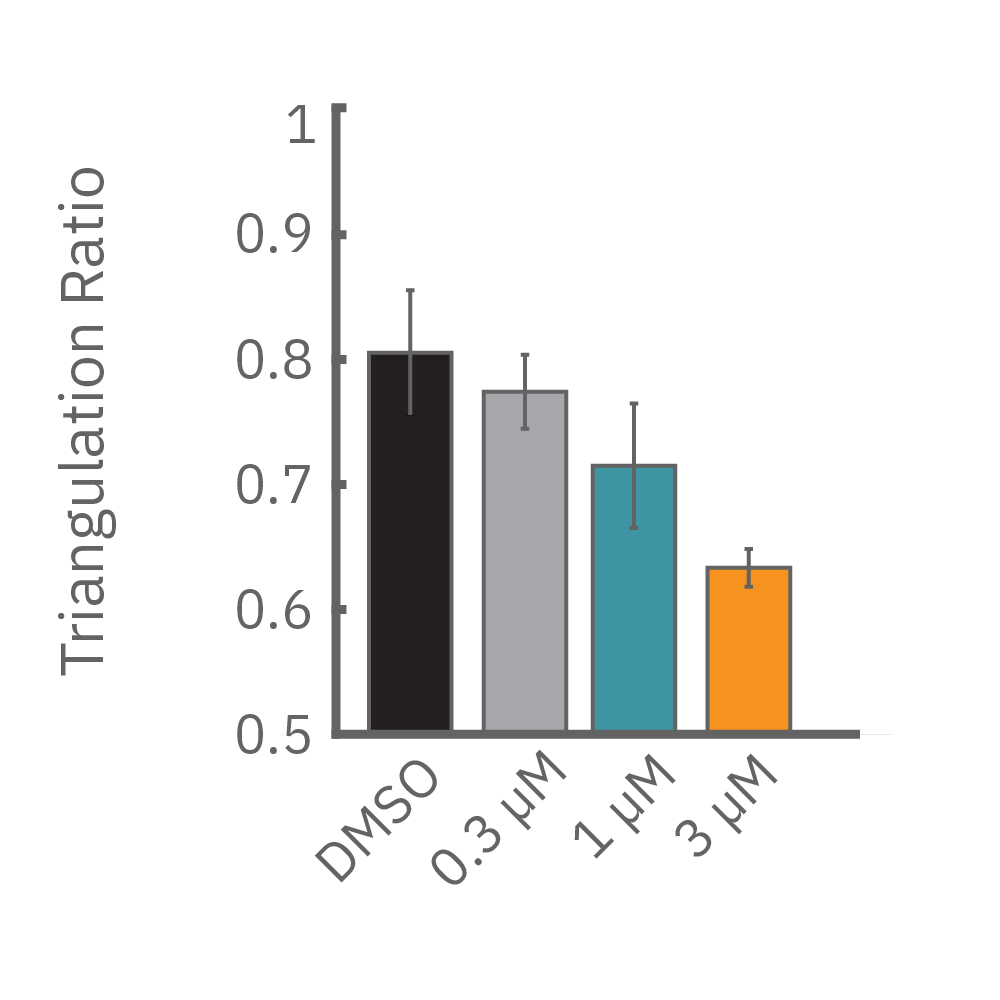
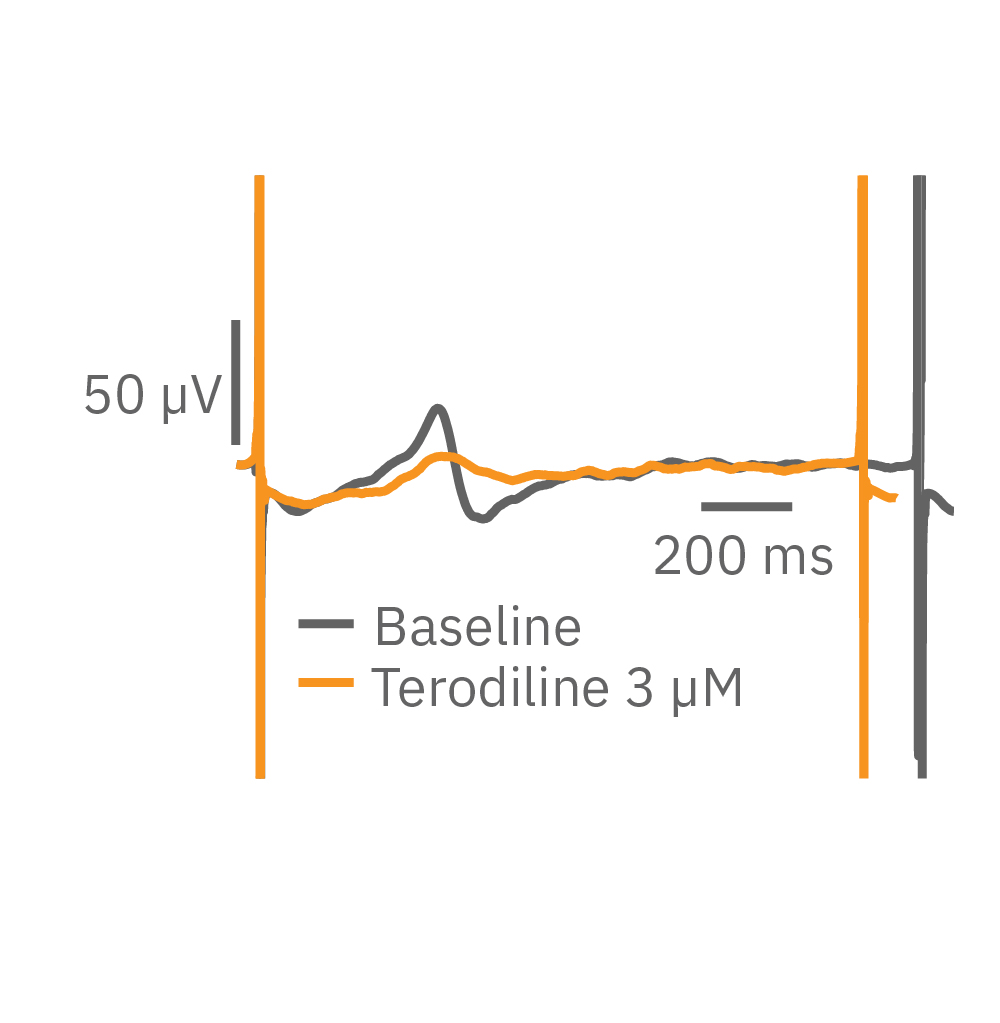
LEAP applications
LEAP is ideal for capturing even small differences in action potential morphology in cardiac health and disease and in response to compounds. LEAP is a powerful tool for many applications including:
- Automated APD and EAD detection for high throughput drug screening
- Predicting arrhythmic risk for cardiac safety and cardiotoxicity testing
- Characterization of action potential morphology in human induced pluripotent stem cell-derived (hiPSC) cardiomycoytes
- Studying the effects of genetic manipulation on cardiac electrophysiology
- Comparing cardiac biology in healthy and diseased states